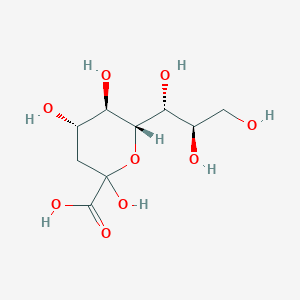
Keto-Deoxy-Nonulonic acid
Overview
Description
3-Deoxy-D-glycero-D-galacto-2-nonulosonic acid is a deaminated sialic acid, a type of sugar acid that plays a crucial role in various biological processes. It was first identified in the glycoproteins of rainbow trout eggs and is known for its presence at the non-reducing ends of oligosialyl chains . This compound is significant in cellular functions such as cell adhesion, signal recognition, and tumor progression .
Mechanism of Action
Target of Action
2-Keto-3-deoxy-nononic acid, also known as 3-Deoxy-D-Glycero-D-Galacto-Non-2-ulopyranosonic Acid or KDN, is a member of the sialic acid family . It is found in almost all types of glycoconjugates, including glycolipids, glycoproteins, and capsular polysaccharides . KDN residues are linked to almost all glycan structures in place of Neu5Ac .
Mode of Action
KDN interacts with its targets through its residues, which are linked to almost all glycan structures . All linkage types known for Neu5Ac; α2,3-, α2,4-, α2,6-, and α2,8- are also found for KDN .
Biochemical Pathways
KDN is biosynthesized de novo using mannose as a precursor sugar, which is activated to CMP-KDN and transferred to acceptor sugar residues . These reactions are catalyzed by enzymes, some of which preferably recognize KDN, but many others prefer Neu5Ac to KDN . The pathway consists of the following three sequential reactions :
Pharmacokinetics
It is known that kdn is biosynthesized de novo in the body .
Result of Action
KDN plays a significant role in cellular recognition and protein-carbohydrate interactions . It is also known to influence the biological properties of a cell . Elevated expression of KDN was found in fetal human red blood cells compared with adult red blood cells, and ovarian tumor tissues compared with normal controls .
Action Environment
The action of KDN can be influenced by various environmental factors. For instance, in human neuroblastoma, nephroblastoma, and breast cancer, short-chain KDN through α2,8 linkages forms polysialic acid, which can weaken cell-cell adhesion and interactions between cells and the matrix, thereby promoting cancer cell separation, infiltration, and distant metastasis .
Biochemical Analysis
Biochemical Properties
2-Keto-3-Deoxy-Nononic Acid is found in almost all types of glycoconjugates, including glycolipids, glycoproteins, and capsular polysaccharides . It interacts with various enzymes, some of which preferably recognize 2-Keto-3-Deoxy-Nononic Acid, but many others prefer N-acetylneuraminic acid to 2-Keto-3-Deoxy-Nononic Acid .
Cellular Effects
Elevated expression of 2-Keto-3-Deoxy-Nononic Acid was found in fetal human red blood cells compared with adult red blood cells, and ovarian tumor tissues compared with normal controls . It influences cell function by modulating the biological properties of a cell .
Molecular Mechanism
The mechanism of action of 2-Keto-3-Deoxy-Nononic Acid involves its biosynthesis de novo using mannose as a precursor sugar, which is activated to CMP-2-Keto-3-Deoxy-Nononic Acid and transferred to acceptor sugar residues .
Metabolic Pathways
2-Keto-3-Deoxy-Nononic Acid is involved in the amino sugar and nucleotide sugar metabolism pathway . It interacts with enzymes and cofactors in this pathway, potentially affecting metabolic flux or metabolite levels .
Transport and Distribution
Given its presence in various types of glycoconjugates, it’s likely that it interacts with transporters or binding proteins, influencing its localization or accumulation .
Preparation Methods
Synthetic Routes and Reaction Conditions
The synthesis of 3-Deoxy-D-glycero-D-galacto-2-nonulosonic acid can be achieved through a multi-step process. One notable method involves the conversion of enantiopure cis-1,2-dihydrocatechol, obtained by microbial oxidation of chlorobenzene, into the target compound via a ten-step reaction sequence . This process includes several key steps such as oxidation, reduction, and protection-deprotection cycles to achieve the desired stereochemistry and functional groups.
Industrial Production Methods
Industrial production methods for 3-Deoxy-D-glycero-D-galacto-2-nonulosonic acid are less documented, but they likely involve similar synthetic routes with optimizations for scale-up. These optimizations may include the use of more efficient catalysts, improved reaction conditions, and streamlined purification processes to enhance yield and reduce costs.
Chemical Reactions Analysis
Types of Reactions
3-Deoxy-D-glycero-D-galacto-2-nonulosonic acid undergoes various chemical reactions, including:
Oxidation: This reaction can modify the hydroxyl groups present in the molecule.
Reduction: Reduction reactions can target the carbonyl groups to form alcohols.
Substitution: Functional groups in the molecule can be substituted with other groups under appropriate conditions.
Common Reagents and Conditions
Common reagents used in these reactions include oxidizing agents like potassium permanganate, reducing agents such as sodium borohydride, and various acids and bases for substitution reactions. The conditions typically involve controlled temperatures and pH to ensure the desired reaction pathway and product formation.
Major Products Formed
The major products formed from these reactions depend on the specific conditions and reagents used For example, oxidation can lead to the formation of carboxylic acids, while reduction can yield alcohol derivatives
Scientific Research Applications
3-Deoxy-D-glycero-D-galacto-2-nonulosonic acid has a wide range of scientific research applications:
Comparison with Similar Compounds
3-Deoxy-D-glycero-D-galacto-2-nonulosonic acid is unique among sialic acids due to its deaminated structure. Similar compounds include:
N-Acetylneuraminic acid: The most common sialic acid, involved in many biological processes.
N-Glycolylneuraminic acid: Another sialic acid variant with a hydroxyl group replacing the acetyl group.
2-Keto-3-deoxy-D-glycero-D-galacto-nononic acid: A structurally similar compound with different functional groups.
Biological Activity
Keto-Deoxy-Nonulonic Acid (KDN), chemically known as 2-keto-3-deoxy-D-glycero-D-galacto-nonulosonic acid, is a sialic acid derivative that plays significant roles in various biological processes. This article explores the biological activity of KDN, focusing on its synthesis, occurrence in different organisms, and implications in host-pathogen interactions.
Synthesis and Occurrence
KDN is synthesized through enzymatic pathways involving specific synthases. The human Neu5Ac-9-P synthase is capable of catalyzing the formation of both KDN-9-P and Neu5Ac-9-P from phosphoenolpyruvate (PEP) and sugar substrates, such as N-acetylmannosamine 6-phosphate (ManNAc-6-P) . This enzymatic activity highlights the biochemical versatility of KDN in mammalian systems.
KDN has been detected in various biological contexts, including glycoproteins and glycolipids from rat tissues and human lung cancer cell lines. Advanced techniques such as high-performance liquid chromatography (HPLC) have confirmed the presence of KDN residues, indicating its relevance in mammalian biology . Moreover, KDN is predominantly found in certain strains of bacteria, particularly Serratia marcescens, where it contributes to the composition of capsular polysaccharides (CPS) .
Biological Functions
1. Role in Pathogenesis:
KDN's presence in bacterial capsules enhances virulence by providing a mechanism for evading host immune responses. For instance, strains of S. marcescens producing KDN exhibited increased resistance to phagocytosis by immune cells, facilitating bacterial survival during infections . The capsule's composition, including KDN, significantly influences the strain's ability to colonize organs such as the spleen and liver during bloodstream infections .
2. Interaction with Host Immune System:
KDN's structural similarity to other sialic acids allows it to mimic host glycoproteins, aiding bacteria in escaping immune detection. This mimicry can lead to reduced opsonization and phagocytosis by macrophages, which is critical for bacterial persistence in host tissues .
Research Findings
Recent studies have highlighted the importance of KDN in various experimental models:
- Capsule-dependent Virulence: In murine models of bacteremia, strains with KDN-rich capsules demonstrated significantly higher survival rates compared to acapsular mutants. This suggests that KDN plays a crucial role in enhancing bacterial fitness during systemic infections .
- Immunological Evasion: Disruption of genes responsible for KDN biosynthesis resulted in increased susceptibility to phagocytosis, indicating that KDN contributes to the protective effects of CPS against host immune mechanisms .
Case Studies
Properties
IUPAC Name |
(4S,5R,6R)-2,4,5-trihydroxy-6-[(1R,2R)-1,2,3-trihydroxypropyl]oxane-2-carboxylic acid | |
---|---|---|
Source | PubChem | |
URL | https://pubchem.ncbi.nlm.nih.gov | |
Description | Data deposited in or computed by PubChem | |
InChI |
InChI=1S/C9H16O9/c10-2-4(12)6(14)7-5(13)3(11)1-9(17,18-7)8(15)16/h3-7,10-14,17H,1-2H2,(H,15,16)/t3-,4+,5+,6+,7+,9?/m0/s1 | |
Source | PubChem | |
URL | https://pubchem.ncbi.nlm.nih.gov | |
Description | Data deposited in or computed by PubChem | |
InChI Key |
CLRLHXKNIYJWAW-QBTAGHCHSA-N | |
Source | PubChem | |
URL | https://pubchem.ncbi.nlm.nih.gov | |
Description | Data deposited in or computed by PubChem | |
Canonical SMILES |
C1C(C(C(OC1(C(=O)O)O)C(C(CO)O)O)O)O | |
Source | PubChem | |
URL | https://pubchem.ncbi.nlm.nih.gov | |
Description | Data deposited in or computed by PubChem | |
Isomeric SMILES |
C1[C@@H]([C@H]([C@@H](OC1(C(=O)O)O)[C@@H]([C@@H](CO)O)O)O)O | |
Source | PubChem | |
URL | https://pubchem.ncbi.nlm.nih.gov | |
Description | Data deposited in or computed by PubChem | |
Molecular Formula |
C9H16O9 | |
Source | PubChem | |
URL | https://pubchem.ncbi.nlm.nih.gov | |
Description | Data deposited in or computed by PubChem | |
DSSTOX Substance ID |
DTXSID50661882 | |
Record name | 3-Deoxy-D-glycero-D-galacto-2-nonulopyranosonic acid | |
Source | EPA DSSTox | |
URL | https://comptox.epa.gov/dashboard/DTXSID50661882 | |
Description | DSSTox provides a high quality public chemistry resource for supporting improved predictive toxicology. | |
Molecular Weight |
268.22 g/mol | |
Source | PubChem | |
URL | https://pubchem.ncbi.nlm.nih.gov | |
Description | Data deposited in or computed by PubChem | |
CAS No. |
153666-19-4 | |
Record name | 3-Deoxy-D-glycero-D-galacto-2-nonulopyranosonic acid | |
Source | EPA DSSTox | |
URL | https://comptox.epa.gov/dashboard/DTXSID50661882 | |
Description | DSSTox provides a high quality public chemistry resource for supporting improved predictive toxicology. | |
Retrosynthesis Analysis
AI-Powered Synthesis Planning: Our tool employs the Template_relevance Pistachio, Template_relevance Bkms_metabolic, Template_relevance Pistachio_ringbreaker, Template_relevance Reaxys, Template_relevance Reaxys_biocatalysis model, leveraging a vast database of chemical reactions to predict feasible synthetic routes.
One-Step Synthesis Focus: Specifically designed for one-step synthesis, it provides concise and direct routes for your target compounds, streamlining the synthesis process.
Accurate Predictions: Utilizing the extensive PISTACHIO, BKMS_METABOLIC, PISTACHIO_RINGBREAKER, REAXYS, REAXYS_BIOCATALYSIS database, our tool offers high-accuracy predictions, reflecting the latest in chemical research and data.
Strategy Settings
Precursor scoring | Relevance Heuristic |
---|---|
Min. plausibility | 0.01 |
Model | Template_relevance |
Template Set | Pistachio/Bkms_metabolic/Pistachio_ringbreaker/Reaxys/Reaxys_biocatalysis |
Top-N result to add to graph | 6 |
Feasible Synthetic Routes
Q1: What is the molecular formula and weight of KDN?
A1: The molecular formula of KDN is C9H16O10, and its molecular weight is 268.22 g/mol.
Q2: What spectroscopic data is available for characterizing KDN?
A2: Researchers commonly utilize techniques like 1H-NMR, 13C-NMR, and circular dichroism (CD) spectral analysis to determine the structure and stereochemistry of KDN and its derivatives. [, , ]
Q3: Are there different methods to synthesize KDN?
A3: Yes, KDN can be synthesized through various methods:
- Base-catalyzed condensation: This approach efficiently yields KDN. []
- Condensation of oxalacetic acid with D-mannose: This method is followed by several steps to obtain the desired KDN derivative. []
- Horner-Wittig reactions: This pathway, utilizing specific starting materials, leads to the synthesis of KDN derivatives. []
- Desymmetrization by ring-closing metathesis: This method enables the introduction of oxygen functionality in a stereocontrolled manner. []
Q4: Can KDN be synthesized from a non-carbohydrate source?
A4: Yes, the first total synthesis of (+)-KDN was achieved using enantiopure cis-1,2-dihydrocatechol, a product obtained by microbial oxidation of chlorobenzene. [, ]
Q5: Where is KDN found in nature?
A5: KDN is found in a variety of organisms, including:
- Fish: Notably, the liver of the loach (Misgurnus fossilis) contains a sialidase enzyme (KDNase) that specifically cleaves KDN. [, ]
- Bacteria: Certain plant pathogenic Streptomyces species incorporate KDN into their cell wall polymers. [, ]
- Mammals: KDN has been detected in various rat organs and tissues, with notable age-related variations observed in the liver. [, ]
- Microalgae: The haptophyte Prymnesium parvum has been found to possess a KDN biosynthesis pathway. []
Q6: What are the biological roles of KDN?
A6: While research on KDN function is ongoing, some insights include:
- Cell wall component: In plant pathogenic Streptomyces, KDN-containing polymers may contribute to the attachment of these bacteria to host plant cells. []
- Potential role in mammals: Studies suggest that KDN biosynthesis might help regulate physiological mannose levels in mammals. Additionally, under specific conditions, KDN-containing glycans could elicit immune responses. []
Q7: What are some important derivatives and analogs of KDN?
A7: Several derivatives and analogs of KDN have been synthesized, including:
- Aryl-α-glycosides: These derivatives have been synthesized and characterized using NMR and CD spectral analysis. []
- Nucleoside analogues: Mono- and disaccharide nucleoside analogues have been synthesized using various reaction conditions, their stereochemistry investigated using NMR. [, ]
- α-N-Glycosides with nucleobases: These derivatives, synthesized using uracil, thymine, and other nucleobases, have been studied for their photocycloaddition properties. [, ]
- Phosphonic acid analogues: These synthetic analogs have shown moderate activity as potential sialidase inhibitors. []
- 4-acylamino-2,6-anhydro-2,3,4-trideoxy-D-glycero-D-galacto-non-2-enoic acids: These compounds, synthesized from KDN, have been structurally characterized using techniques like NMR and X-ray crystallography. [, ]
- Ganglioside GM4 and GM3 analogs: These synthetic analogs, containing KDN in place of N-acetylneuraminic acid, have been successfully produced. []
- Tetrazolyl derivatives: These have been explored as useful glycosyl donors for synthesizing O- and C-glycosides. []
Q8: How does the structure of KDN impact its activity?
A8: While specific structure-activity relationships require further investigation, some modifications have demonstrated effects:
- Gangliosides: Studies on chemically synthesized GM3 and GM4, containing KDN, showed that the length of the fatty acyl chain inversely correlates with immunosuppressive activity. Modifications like hydroxylation of the fatty acyl group can decrease immunosuppressive activity. []
- Sialidase inhibition: Replacing the carboxyl group of KDN with a phosphonic acid group resulted in moderate sialidase inhibitory activity. []
Q9: How is KDN typically analyzed and quantified?
A9: Several analytical methods are employed for KDN:
- High-performance liquid chromatography (HPLC): This technique, particularly with a strongly basic anion-exchange resin, is used to analyze and isolate KDN. []
- HPLC with pulsed amperometric detection (PAD): This method allows simultaneous analysis of KDN alongside other sialic acids like Neu5Ac and Neu5Gc. []
- Gas chromatography/mass spectrometry (GC/MS): This technique is valuable for characterizing KDN and other sialic acid-like monosaccharides. The method involves converting KDN to its acetylated lactone form prior to analysis. []
Q10: Does KDN have any specific applications?
A10: Research on KDN applications is ongoing, but some potential areas include:
- Glycoscience research: KDN and its derivatives are valuable tools for studying sialic acid biology and enzymology. [, ]
- Molecular probes: Synthetic KDN derivatives can be used to investigate the substrate specificity of enzymes like human α1,3-fucosyltransferases. []
- Potential therapeutic targets: While still under investigation, KDN-related pathways could be explored for potential therapeutic interventions, particularly in cases where KDN levels are elevated. []
Disclaimer and Information on In-Vitro Research Products
Please be aware that all articles and product information presented on BenchChem are intended solely for informational purposes. The products available for purchase on BenchChem are specifically designed for in-vitro studies, which are conducted outside of living organisms. In-vitro studies, derived from the Latin term "in glass," involve experiments performed in controlled laboratory settings using cells or tissues. It is important to note that these products are not categorized as medicines or drugs, and they have not received approval from the FDA for the prevention, treatment, or cure of any medical condition, ailment, or disease. We must emphasize that any form of bodily introduction of these products into humans or animals is strictly prohibited by law. It is essential to adhere to these guidelines to ensure compliance with legal and ethical standards in research and experimentation.