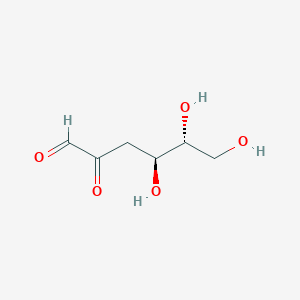
3-Deoxyglucosone
Overview
Description
3-Deoxy Glucosone is a highly reactive dicarbonyl sugar, notable for its role as a marker for diabetes. It is formed through the Maillard reaction, where glucose reacts with primary amino groups of lysine or arginine found in proteins. This compound is significant due to its involvement in the formation of advanced glycation end-products (AGEs), which contribute to various diseases such as diabetes, atherosclerosis, hypertension, Alzheimer’s disease, inflammation, and aging .
Mechanism of Action
Target of Action
3-Deoxyglucosone (3-DG) primarily targets proteins, particularly those containing lysine or arginine residues . It reacts with these amino groups to initiate the glycation process . The glycation of proteins can compromise their structure and functionality, leading to various complications .
Mode of Action
3-DG is a powerful glycating agent that reacts with proteins to form Advanced Glycation End-products (AGEs) . As a dicarbonyl sugar, 3-DG is highly reactive towards amine groups, which are common in amino acids and some nucleic acids . The products from the reaction of 3-DG with protein amino groups are called AGEs, which include imidazolones, pyrraline, N6-(carboxymethyl)lysine, and pentosidine .
Biochemical Pathways
3-DG is produced via the Maillard reaction, a non-enzymatic glycation reaction between reducing sugars and primary amino groups in biomolecules . It forms after glucose reacts with primary amino groups of lysine or arginine found in proteins . The formation of 3-DG is significantly elevated during diabetes, leading to vascular damage through the glycation reaction . 3-DG also arises via the degradation of fructose 3-phosphate (F3P) .
Pharmacokinetics
It is known that the production of 3-dg is significantly elevated during diabetes, suggesting that its concentration in the body can be influenced by metabolic conditions .
Result of Action
The formation of AGEs by 3-DG can lead to the modification and cross-linking of long-lived proteins such as crystallin and collagen . This contributes to diseases such as the vascular complications of diabetes, atherosclerosis, hypertension, Alzheimer’s disease, inflammation, and aging . In addition, 3-DG has been found to interfere with insulin signaling and attenuate insulin action on glucose-induced GLP-1 secretion in the enteroendocrine L cell line STC-1 .
Action Environment
Environmental factors such as diet, physical activity, stress, and pollution can influence the formation of AGEs . For instance, a hyperglycemic environment, as seen in diabetes, can accelerate the formation of AGEs . Furthermore, oxidative stress, which can be induced by various environmental factors, promotes the formation of α-dicarbonyls or α-oxoaldehydes, including 3-DG .
Biochemical Analysis
Biochemical Properties
3-Deoxyglucosone is a potent glycating agent that interacts with various biomolecules, leading to the formation of AGEs. It is highly reactive towards amine groups found in amino acids and nucleic acids. The compound is produced through the degradation of fructose-3-phosphate and the Maillard reaction involving glucose and amino groups. Enzymes such as fructosamine-3-kinase play a role in its formation. The interaction of this compound with proteins results in structural modifications and cross-linking, contributing to diseases like diabetes, atherosclerosis, and Alzheimer’s disease .
Cellular Effects
This compound has profound effects on various cell types and cellular processes. It induces oxidative stress and carbonyl stress, leading to cellular damage. The compound influences cell signaling pathways, gene expression, and cellular metabolism. For instance, it has been shown to modify histone proteins, affecting chromatin structure and gene expression. Elevated levels of this compound are associated with diabetic complications, where it contributes to vascular damage and inflammation .
Molecular Mechanism
At the molecular level, this compound exerts its effects through glycation reactions. It forms Schiff bases and Amadori products with amino groups in proteins, leading to the formation of AGEs. These AGEs can alter protein structure and function, inhibit enzyme activity, and affect cellular signaling. The compound’s interaction with histone proteins, for example, results in structural changes that compromise chromatin integrity and function .
Temporal Effects in Laboratory Settings
In laboratory settings, the effects of this compound change over time. The compound is relatively stable but can degrade into other reactive intermediates. Long-term exposure to this compound in vitro and in vivo studies has shown persistent oxidative stress and protein modification, leading to chronic cellular dysfunction. These effects are particularly pronounced in diabetic models, where prolonged exposure exacerbates complications .
Dosage Effects in Animal Models
The effects of this compound vary with different dosages in animal models. Low doses may induce mild oxidative stress and protein glycation, while high doses can lead to significant cellular damage and toxicity. In diabetic animal models, elevated levels of this compound correlate with increased severity of complications such as nephropathy and retinopathy. Toxic effects at high doses include impaired immune response and vascular damage .
Metabolic Pathways
This compound is involved in several metabolic pathways. It is produced from fructose-3-phosphate via the polyol pathway and through the Maillard reaction involving glucose. Enzymes such as fructosamine-3-kinase and aldose reductase are key players in its metabolism. The compound’s presence affects metabolic flux and can lead to the accumulation of AGEs, which further disrupts cellular homeostasis .
Transport and Distribution
Within cells and tissues, this compound is transported and distributed through interactions with various transporters and binding proteins. It can accumulate in tissues with high metabolic activity, such as the liver and kidneys. The compound’s distribution is influenced by its reactivity and the presence of target biomolecules. In diabetic conditions, its accumulation is more pronounced, contributing to tissue-specific complications .
Subcellular Localization
This compound’s subcellular localization is determined by its interactions with cellular components. It can be found in the cytoplasm, where it interacts with proteins and nucleic acids. The compound’s reactivity allows it to modify proteins in various cellular compartments, including the nucleus, where it affects histone proteins and chromatin structure. Post-translational modifications and targeting signals may direct its localization to specific organelles .
Preparation Methods
Synthetic Routes and Reaction Conditions: 3-Deoxy Glucosone is naturally produced via the Maillard reaction, which involves the non-enzymatic reaction of glucose with amino groups in proteins. This reaction is accelerated under conditions of high glucose concentration, such as in uncontrolled diabetes .
Industrial Production Methods: Industrial production of 3-Deoxy Glucosone typically involves the controlled Maillard reaction, where glucose is reacted with amino acids under specific conditions to yield the desired compound. The reaction conditions, such as temperature and pH, are carefully monitored to optimize the yield and purity of 3-Deoxy Glucosone .
Chemical Reactions Analysis
Types of Reactions: 3-Deoxy Glucosone undergoes several types of chemical reactions, including:
Oxidation: It can be oxidized to form various oxidation products.
Reduction: It can be reduced to form corresponding alcohols.
Substitution: It can react with nucleophiles to form substituted products.
Common Reagents and Conditions:
Oxidation: Common oxidizing agents include hydrogen peroxide and potassium permanganate.
Reduction: Common reducing agents include sodium borohydride and lithium aluminum hydride.
Substitution: Nucleophiles such as amines and thiols are commonly used in substitution reactions.
Major Products Formed: The major products formed from these reactions include advanced glycation end-products (AGEs) such as imidazolones, pyrraline, N6-(carboxymethyl)lysine, and pentosidine .
Scientific Research Applications
3-Deoxy Glucosone has a wide range of scientific research applications, including:
Comparison with Similar Compounds
Methylglyoxal: Another reactive dicarbonyl compound involved in the formation of AGEs.
Glyoxal: A simpler dicarbonyl compound that also forms AGEs.
Fructosamine: A compound formed from the reaction of glucose with amino groups in proteins, similar to 3-Deoxy Glucosone.
Uniqueness: 3-Deoxy Glucosone is unique due to its specific formation pathway via the Maillard reaction and its significant role in the formation of AGEs. Its elevated levels in diabetic conditions make it a valuable biomarker for diabetes and its complications .
Biological Activity
3-Deoxyglucosone (3-DG) is a dicarbonyl compound that plays a significant role in the glycation process, leading to the formation of advanced glycation end products (AGEs). These AGEs are implicated in various pathological conditions, particularly in diabetes and its complications. This article reviews the biological activity of 3-DG, focusing on its effects on cellular mechanisms, metabolic pathways, and potential therapeutic implications.
Overview of this compound
3-DG is formed during the Maillard reaction, a chemical reaction between amino acids and reducing sugars that occurs during food processing and cooking. It is also produced in vivo from glucose metabolism, especially under hyperglycemic conditions. Its reactivity with proteins can lead to significant modifications, impacting protein structure and function.
Glycation and Formation of AGEs
The glycation of proteins by 3-DG results in the formation of AGEs, which are known to contribute to diabetic complications such as nephropathy, retinopathy, and cardiovascular diseases. The mechanism involves the non-enzymatic reaction between 3-DG and amino groups of proteins, leading to structural changes that can disrupt normal cellular functions.
Key Findings on Glycation
- Histone Glycation : A study demonstrated that 3-DG can glycate histone H1 proteins, affecting their secondary structure and potentially compromising chromatin integrity. This alteration may influence gene expression related to diabetic complications .
- AGE Formation : Research indicates that 3-DG is a potent glycating agent compared to other dicarbonyls like methylglyoxal. It reacts rapidly with lysine and arginine residues on proteins, forming AGEs such as Nε-carboxymethyl lysine and pentosidine .
Impact on Intestinal Microbiota
In a study involving prediabetic rats treated with 3-DG, alterations in intestinal microbiota composition were observed. The treatment led to an increase in Proteobacteria abundance while reducing levels of beneficial bacteria. This dysbiosis was associated with impaired glucose metabolism and increased levels of lipopolysaccharides (LPS), suggesting a link between dietary 3-DG intake and metabolic disorders .
Cellular Mechanisms
Research has shown that 3-DG influences various cellular pathways:
- Oxidative Stress : 3-DG has been linked to increased oxidative stress in cells, contributing to apoptosis through the activation of caspases .
- Insulin Resistance : Elevated levels of 3-DG have been correlated with insulin resistance in diabetic models. It affects insulin signaling pathways by modifying key proteins involved in glucose metabolism .
Case Studies
- Prediabetes Model : In a controlled study on rats induced with prediabetes through high-fat diets, administration of 50 mg/kg of 3-DG for two weeks resulted in significant metabolic dysfunctions. The study highlighted changes in plasma GLP-2 levels and increased fasting glucose levels, linking dietary 3-DG to prediabetic conditions .
- Histone Modification : Another study focused on the effects of 3-DG on histone proteins indicated that glycation by 3-DG could lead to altered gene expression patterns associated with diabetic complications .
Data Summary
Study Focus | Findings | Implications |
---|---|---|
Intestinal Microbiota | Increased Proteobacteria; decreased beneficial bacteria | Links dietary intake of 3-DG to metabolic disorders |
Histone Glycation | Structural changes in H1 histone due to glycation | Potential impacts on gene regulation |
Metabolic Dysfunction | Impaired glucose tolerance and increased LPS levels | Suggests role in development of prediabetes |
Properties
IUPAC Name |
(4S,5R)-4,5,6-trihydroxy-2-oxohexanal | |
---|---|---|
Source | PubChem | |
URL | https://pubchem.ncbi.nlm.nih.gov | |
Description | Data deposited in or computed by PubChem | |
InChI |
InChI=1S/C6H10O5/c7-2-4(9)1-5(10)6(11)3-8/h2,5-6,8,10-11H,1,3H2/t5-,6+/m0/s1 | |
Source | PubChem | |
URL | https://pubchem.ncbi.nlm.nih.gov | |
Description | Data deposited in or computed by PubChem | |
InChI Key |
ZGCHLOWZNKRZSN-NTSWFWBYSA-N | |
Source | PubChem | |
URL | https://pubchem.ncbi.nlm.nih.gov | |
Description | Data deposited in or computed by PubChem | |
Canonical SMILES |
C(C(C(CO)O)O)C(=O)C=O | |
Source | PubChem | |
URL | https://pubchem.ncbi.nlm.nih.gov | |
Description | Data deposited in or computed by PubChem | |
Isomeric SMILES |
C([C@@H]([C@@H](CO)O)O)C(=O)C=O | |
Source | PubChem | |
URL | https://pubchem.ncbi.nlm.nih.gov | |
Description | Data deposited in or computed by PubChem | |
Molecular Formula |
C6H10O5 | |
Source | PubChem | |
URL | https://pubchem.ncbi.nlm.nih.gov | |
Description | Data deposited in or computed by PubChem | |
DSSTOX Substance ID |
DTXSID00193820 | |
Record name | 3-Deoxyglucosone | |
Source | EPA DSSTox | |
URL | https://comptox.epa.gov/dashboard/DTXSID00193820 | |
Description | DSSTox provides a high quality public chemistry resource for supporting improved predictive toxicology. | |
Molecular Weight |
162.14 g/mol | |
Source | PubChem | |
URL | https://pubchem.ncbi.nlm.nih.gov | |
Description | Data deposited in or computed by PubChem | |
CAS No. |
4084-27-9, 30382-30-0 | |
Record name | 3-Deoxyglucosone | |
Source | CAS Common Chemistry | |
URL | https://commonchemistry.cas.org/detail?cas_rn=4084-27-9 | |
Description | CAS Common Chemistry is an open community resource for accessing chemical information. Nearly 500,000 chemical substances from CAS REGISTRY cover areas of community interest, including common and frequently regulated chemicals, and those relevant to high school and undergraduate chemistry classes. This chemical information, curated by our expert scientists, is provided in alignment with our mission as a division of the American Chemical Society. | |
Explanation | The data from CAS Common Chemistry is provided under a CC-BY-NC 4.0 license, unless otherwise stated. | |
Record name | 3-Deoxyglucosone | |
Source | ChemIDplus | |
URL | https://pubchem.ncbi.nlm.nih.gov/substance/?source=chemidplus&sourceid=0004084279 | |
Description | ChemIDplus is a free, web search system that provides access to the structure and nomenclature authority files used for the identification of chemical substances cited in National Library of Medicine (NLM) databases, including the TOXNET system. | |
Record name | erythro-Hexosulose, 3-deoxy- | |
Source | ChemIDplus | |
URL | https://pubchem.ncbi.nlm.nih.gov/substance/?source=chemidplus&sourceid=0030382300 | |
Description | ChemIDplus is a free, web search system that provides access to the structure and nomenclature authority files used for the identification of chemical substances cited in National Library of Medicine (NLM) databases, including the TOXNET system. | |
Record name | 3-Deoxyglucosone | |
Source | EPA DSSTox | |
URL | https://comptox.epa.gov/dashboard/DTXSID00193820 | |
Description | DSSTox provides a high quality public chemistry resource for supporting improved predictive toxicology. | |
Record name | 3-Deoxy-D-glucosone | |
Source | European Chemicals Agency (ECHA) | |
URL | https://echa.europa.eu/information-on-chemicals | |
Description | The European Chemicals Agency (ECHA) is an agency of the European Union which is the driving force among regulatory authorities in implementing the EU's groundbreaking chemicals legislation for the benefit of human health and the environment as well as for innovation and competitiveness. | |
Explanation | Use of the information, documents and data from the ECHA website is subject to the terms and conditions of this Legal Notice, and subject to other binding limitations provided for under applicable law, the information, documents and data made available on the ECHA website may be reproduced, distributed and/or used, totally or in part, for non-commercial purposes provided that ECHA is acknowledged as the source: "Source: European Chemicals Agency, http://echa.europa.eu/". Such acknowledgement must be included in each copy of the material. ECHA permits and encourages organisations and individuals to create links to the ECHA website under the following cumulative conditions: Links can only be made to webpages that provide a link to the Legal Notice page. | |
Record name | 3-DEOXYGLUCOSONE | |
Source | FDA Global Substance Registration System (GSRS) | |
URL | https://gsrs.ncats.nih.gov/ginas/app/beta/substances/EXV5374VEY | |
Description | The FDA Global Substance Registration System (GSRS) enables the efficient and accurate exchange of information on what substances are in regulated products. Instead of relying on names, which vary across regulatory domains, countries, and regions, the GSRS knowledge base makes it possible for substances to be defined by standardized, scientific descriptions. | |
Explanation | Unless otherwise noted, the contents of the FDA website (www.fda.gov), both text and graphics, are not copyrighted. They are in the public domain and may be republished, reprinted and otherwise used freely by anyone without the need to obtain permission from FDA. Credit to the U.S. Food and Drug Administration as the source is appreciated but not required. | |
Retrosynthesis Analysis
AI-Powered Synthesis Planning: Our tool employs the Template_relevance Pistachio, Template_relevance Bkms_metabolic, Template_relevance Pistachio_ringbreaker, Template_relevance Reaxys, Template_relevance Reaxys_biocatalysis model, leveraging a vast database of chemical reactions to predict feasible synthetic routes.
One-Step Synthesis Focus: Specifically designed for one-step synthesis, it provides concise and direct routes for your target compounds, streamlining the synthesis process.
Accurate Predictions: Utilizing the extensive PISTACHIO, BKMS_METABOLIC, PISTACHIO_RINGBREAKER, REAXYS, REAXYS_BIOCATALYSIS database, our tool offers high-accuracy predictions, reflecting the latest in chemical research and data.
Strategy Settings
Precursor scoring | Relevance Heuristic |
---|---|
Min. plausibility | 0.01 |
Model | Template_relevance |
Template Set | Pistachio/Bkms_metabolic/Pistachio_ringbreaker/Reaxys/Reaxys_biocatalysis |
Top-N result to add to graph | 6 |
Feasible Synthetic Routes
Disclaimer and Information on In-Vitro Research Products
Please be aware that all articles and product information presented on BenchChem are intended solely for informational purposes. The products available for purchase on BenchChem are specifically designed for in-vitro studies, which are conducted outside of living organisms. In-vitro studies, derived from the Latin term "in glass," involve experiments performed in controlled laboratory settings using cells or tissues. It is important to note that these products are not categorized as medicines or drugs, and they have not received approval from the FDA for the prevention, treatment, or cure of any medical condition, ailment, or disease. We must emphasize that any form of bodily introduction of these products into humans or animals is strictly prohibited by law. It is essential to adhere to these guidelines to ensure compliance with legal and ethical standards in research and experimentation.