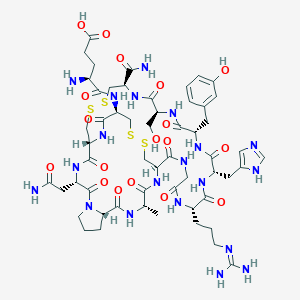
Conotoxin GI
Overview
Description
Alpha-Conotoxin GI is a peptide neurotoxin isolated from the venom of the marine cone snail, Conus geographus. This compound is known for its potent and selective antagonistic activity against nicotinic acetylcholine receptors (nAChRs), specifically targeting the alpha/delta subunit interface . Due to its high specificity and potency, Conotoxin GI has become a valuable tool in neuropharmacological research and has potential therapeutic applications.
Preparation Methods
Synthetic Routes and Reaction Conditions
The chemical synthesis of Conotoxin GI involves solid-phase peptide synthesis (SPPS) techniques. The peptide is assembled step-by-step on a resin support, with each amino acid being added sequentially. The synthesis typically employs Fmoc (9-fluorenylmethyloxycarbonyl) chemistry for temporary protection of the amino groups . After the peptide chain is fully assembled, it is cleaved from the resin and subjected to oxidative folding to form the correct disulfide bonds .
Industrial Production Methods
Industrial production of this compound follows similar principles as laboratory synthesis but on a larger scale. The process involves automated peptide synthesizers to increase efficiency and yield. The oxidative folding step is optimized to ensure the correct formation of disulfide bonds, which is crucial for the biological activity of the peptide .
Chemical Reactions Analysis
Types of Reactions
Alpha-Conotoxin GI primarily undergoes oxidation reactions to form disulfide bonds, which are essential for its three-dimensional structure and biological activity . Additionally, it can undergo substitution reactions where specific amino acids are replaced to create analogs with enhanced stability or activity .
Common Reagents and Conditions
Oxidation: Typically performed using air oxidation or iodine in methanol to form disulfide bonds.
Substitution: Amino acid substitutions are achieved using standard SPPS techniques with modified amino acids.
Major Products Formed
The major products formed from these reactions are the correctly folded Conotoxin GI with its native disulfide bonds and various analogs with modified amino acid sequences .
Scientific Research Applications
Alpha-Conotoxin GI has a wide range of scientific research applications:
Neuropharmacology: Used as a tool to study the function and structure of nicotinic acetylcholine receptors.
Drug Development: Serves as a lead compound for developing new therapeutics targeting nAChRs, which are implicated in various neurological disorders.
Pain Management: Potential therapeutic applications in treating chronic pain due to its ability to block specific nAChR subtypes.
Biochemical Research: Utilized in structure-activity relationship (SAR) studies to understand the interactions between peptides and their receptors.
Mechanism of Action
Alpha-Conotoxin GI exerts its effects by binding to the alpha/delta subunit interface of the nicotinic acetylcholine receptor (nAChR), thereby blocking the receptor’s activity . This binding prevents the normal action of acetylcholine, a neurotransmitter, leading to the inhibition of neuromuscular transmission . The high specificity of Conotoxin GI for the alpha/delta site over the alpha/gamma site makes it a valuable pharmacological tool .
Comparison with Similar Compounds
Alpha-Conotoxin GI is part of a larger family of conotoxins, each with unique properties and specificities:
Alpha-Conotoxin ImI: Targets neuronal nAChRs and has applications in studying neuronal signaling.
Alpha-Conotoxin MII: Selectively inhibits alpha3beta2 nAChRs, useful in addiction research.
Alpha-Conotoxin PnIA: Another conotoxin with specificity for neuronal nAChRs, used in neuropharmacological studies.
Compared to these similar compounds, Conotoxin GI is unique in its high specificity for the muscle-type nAChRs, particularly the alpha/delta subunit interface . This specificity makes it an unparalleled tool for studying neuromuscular transmission and developing therapeutics targeting muscle-type nAChRs.
Properties
IUPAC Name |
(4S)-4-amino-5-[[(1R,7S,10S,13S,16S,19R,24R,27S,33S,36S,43R)-27-(2-amino-2-oxoethyl)-19-carbamoyl-7-[3-(diaminomethylideneamino)propyl]-16-(hydroxymethyl)-13-[(3-hydroxyphenyl)methyl]-10-(1H-imidazol-5-ylmethyl)-36-methyl-2,5,8,11,14,17,25,28,34,37,44-undecaoxo-21,22,40,41-tetrathia-3,6,9,12,15,18,26,29,35,38,45-undecazatricyclo[22.14.7.029,33]pentatetracontan-43-yl]amino]-5-oxopentanoic acid | |
---|---|---|
Source | PubChem | |
URL | https://pubchem.ncbi.nlm.nih.gov | |
Description | Data deposited in or computed by PubChem | |
InChI |
InChI=1S/C55H80N20O18S4/c1-25-44(83)72-36-21-95-97-22-37(73-45(84)29(56)9-10-42(80)81)52(91)74-38(51(90)69-33(16-40(57)78)54(93)75-12-4-8-39(75)53(92)65-25)23-96-94-20-35(43(58)82)71-50(89)34(19-76)70-48(87)31(14-26-5-2-6-28(77)13-26)67-49(88)32(15-27-17-61-24-64-27)68-47(86)30(7-3-11-62-55(59)60)66-41(79)18-63-46(36)85/h2,5-6,13,17,24-25,29-39,76-77H,3-4,7-12,14-16,18-23,56H2,1H3,(H2,57,78)(H2,58,82)(H,61,64)(H,63,85)(H,65,92)(H,66,79)(H,67,88)(H,68,86)(H,69,90)(H,70,87)(H,71,89)(H,72,83)(H,73,84)(H,74,91)(H,80,81)(H4,59,60,62)/t25-,29-,30-,31-,32-,33-,34-,35-,36-,37-,38-,39-/m0/s1 | |
Source | PubChem | |
URL | https://pubchem.ncbi.nlm.nih.gov | |
Description | Data deposited in or computed by PubChem | |
InChI Key |
CSUQNJQRLCOOSR-NAKBKFBQSA-N | |
Source | PubChem | |
URL | https://pubchem.ncbi.nlm.nih.gov | |
Description | Data deposited in or computed by PubChem | |
Canonical SMILES |
CC1C(=O)NC2CSSCC(C(=O)NC(CSSCC(NC(=O)C(NC(=O)C(NC(=O)C(NC(=O)C(NC(=O)CNC2=O)CCCN=C(N)N)CC3=CN=CN3)CC4=CC(=CC=C4)O)CO)C(=O)N)C(=O)NC(C(=O)N5CCCC5C(=O)N1)CC(=O)N)NC(=O)C(CCC(=O)O)N | |
Source | PubChem | |
URL | https://pubchem.ncbi.nlm.nih.gov | |
Description | Data deposited in or computed by PubChem | |
Isomeric SMILES |
C[C@H]1C(=O)N[C@H]2CSSC[C@@H](C(=O)N[C@@H](CSSC[C@H](NC(=O)[C@@H](NC(=O)[C@@H](NC(=O)[C@@H](NC(=O)[C@@H](NC(=O)CNC2=O)CCCN=C(N)N)CC3=CN=CN3)CC4=CC(=CC=C4)O)CO)C(=O)N)C(=O)N[C@H](C(=O)N5CCC[C@H]5C(=O)N1)CC(=O)N)NC(=O)[C@H](CCC(=O)O)N | |
Source | PubChem | |
URL | https://pubchem.ncbi.nlm.nih.gov | |
Description | Data deposited in or computed by PubChem | |
Molecular Formula |
C55H80N20O18S4 | |
Source | PubChem | |
URL | https://pubchem.ncbi.nlm.nih.gov | |
Description | Data deposited in or computed by PubChem | |
Molecular Weight |
1437.6 g/mol | |
Source | PubChem | |
URL | https://pubchem.ncbi.nlm.nih.gov | |
Description | Data deposited in or computed by PubChem | |
CAS No. |
76862-65-2 | |
Record name | Conotoxin GI | |
Source | ChemIDplus | |
URL | https://pubchem.ncbi.nlm.nih.gov/substance/?source=chemidplus&sourceid=0076862652 | |
Description | ChemIDplus is a free, web search system that provides access to the structure and nomenclature authority files used for the identification of chemical substances cited in National Library of Medicine (NLM) databases, including the TOXNET system. | |
Retrosynthesis Analysis
AI-Powered Synthesis Planning: Our tool employs the Template_relevance Pistachio, Template_relevance Bkms_metabolic, Template_relevance Pistachio_ringbreaker, Template_relevance Reaxys, Template_relevance Reaxys_biocatalysis model, leveraging a vast database of chemical reactions to predict feasible synthetic routes.
One-Step Synthesis Focus: Specifically designed for one-step synthesis, it provides concise and direct routes for your target compounds, streamlining the synthesis process.
Accurate Predictions: Utilizing the extensive PISTACHIO, BKMS_METABOLIC, PISTACHIO_RINGBREAKER, REAXYS, REAXYS_BIOCATALYSIS database, our tool offers high-accuracy predictions, reflecting the latest in chemical research and data.
Strategy Settings
Precursor scoring | Relevance Heuristic |
---|---|
Min. plausibility | 0.01 |
Model | Template_relevance |
Template Set | Pistachio/Bkms_metabolic/Pistachio_ringbreaker/Reaxys/Reaxys_biocatalysis |
Top-N result to add to graph | 6 |
Feasible Synthetic Routes
Disclaimer and Information on In-Vitro Research Products
Please be aware that all articles and product information presented on BenchChem are intended solely for informational purposes. The products available for purchase on BenchChem are specifically designed for in-vitro studies, which are conducted outside of living organisms. In-vitro studies, derived from the Latin term "in glass," involve experiments performed in controlled laboratory settings using cells or tissues. It is important to note that these products are not categorized as medicines or drugs, and they have not received approval from the FDA for the prevention, treatment, or cure of any medical condition, ailment, or disease. We must emphasize that any form of bodily introduction of these products into humans or animals is strictly prohibited by law. It is essential to adhere to these guidelines to ensure compliance with legal and ethical standards in research and experimentation.