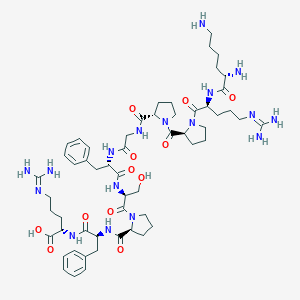
Kallidin
Overview
Description
Scientific Research Applications
Kallidin has a wide range of applications in scientific research, including:
Mechanism of Action
Target of Action
Kallidin is a bioactive kinin that is part of the kallikrein-kinin system (KKS) . The primary targets of this compound are the bradykinin B1 receptor (B1R) and the bradykinin B2 receptor (B2R) . These receptors are involved in various physiological processes such as blood pressure regulation, inflammation, and renal function .
Mode of Action
This compound is produced from kininogen substrates by the action of plasma and tissue kallikreins . Once formed, this compound can be converted to bradykinin by the aminopeptidase enzyme . The active kinin, whether it’s this compound or its derivative bradykinin, interacts with the B1R and B2R . The effects of these interactions are mediated by these receptors, with B2R being constitutively expressed and B1R being inducible during immunopathology .
Biochemical Pathways
The KKS, of which this compound is a part, is involved in a number of crucial physiological processes. These include blood pressure maintenance, hemostasis, inflammation, and renal function . The activation of the KKS, which includes the formation of this compound, can lead to vascular effects (vasodilation, increased microvascular permeability), inflammatory manifestations (edema, pain, increased local blood flow), smooth muscle contraction, and epithelial cell stimulation .
Pharmacokinetics
It is known that this compound is a short-lived peptide , suggesting that it is rapidly metabolized and excreted
Result of Action
The interaction of this compound with its targets leads to a range of molecular and cellular effects. These include vasodilation, increased microvascular permeability, inflammation, and pain . These effects are mediated through the activation of signaling molecules like protein kinase C and phospholipases, and secondary messengers like inositol-1,4,5,-triphosphate, diacylglycerol, calcium, and arachidonic acid .
Action Environment
Environmental factors can influence the action of this compound. For instance, certain environmental contaminants, such as small molecular organic chemicals, engineered nanoparticles, and atmospheric fine particulate matter, can directly interact with the KKS, causing the autoactivation of the Hageman factor XII (FXII), the subsequent cascade cleavage of the plasma prekallikrein (PPK), and high molecular kininogen (HK) . This interaction can lead to downstream hematological effects and other related toxicities .
Biochemical Analysis
Biochemical Properties
Kallidin is produced on cell surfaces by limited proteolysis from their precursors, the kininogens . It is processed by kinin-liberating enzymes named kallikreins . Tissue kallikrein mostly utilizes L-kininogen as the substrate to produce the kinin this compound, whereas plasma kallikrein acts mostly if not exclusively on H-kininogen to generate the bradykinin hormone .
Cellular Effects
This compound plays a significant role in various cellular processes. It acts as a vasodilator and an inflammatory mediator in various signaling cascades . It is associated with inflammatory response pathway mediating diverse functions in vascular permeability like thrombosis and blood coagulation .
Molecular Mechanism
This compound exerts its effects at the molecular level through its interactions with various biomolecules. It binds to G-protein-coupled receptors and triggers Ca2±dependent nitric oxide and/or prostaglandin-dependent signaling pathways .
Temporal Effects in Laboratory Settings
It is known that this compound is a short-lived peptide hormone , indicating that its effects may be transient and could change over time.
Metabolic Pathways
This compound is involved in the kallikrein–kinin system (KKS), a metabolic pathway associated with inflammatory response . It interacts with various enzymes and cofactors within this pathway .
Preparation Methods
Synthetic Routes and Reaction Conditions: Kallidin is produced by the proteolytic cleavage of kininogen through the action of tissue kallikrein . The process involves the cleavage of low-molecular-weight kininogen to produce this compound. This reaction typically occurs under physiological conditions, with tissue kallikrein acting as the enzyme.
Industrial Production Methods: In an industrial setting, this compound can be synthesized using recombinant DNA technology to produce tissue kallikrein, which is then used to cleave kininogen to produce this compound. This method ensures a high yield and purity of the compound .
Chemical Reactions Analysis
Types of Reactions: Kallidin undergoes several types of reactions, including:
Proteolytic Cleavage: this compound can be converted to bradykinin by aminopeptidase.
Degradation: this compound can be degraded by carboxypeptidase M and N, as well as other peptidases.
Common Reagents and Conditions:
Aminopeptidase Enzyme: Converts this compound to bradykinin.
Carboxypeptidase M and N: Degrade this compound into smaller peptides.
Major Products:
Bradykinin: Formed by the action of aminopeptidase on this compound.
Des-Arg10-Kallidin: Formed by the action of carboxypeptidase.
Comparison with Similar Compounds
Bradykinin: A nonapeptide that is very similar to kallidin but lacks the lysine residue at the N-terminal end.
Des-Arg9-Bradykinin: A metabolite of bradykinin that has lost the C-terminal arginine residue.
Des-Arg10-Kallidin: A metabolite of this compound that has lost the C-terminal arginine residue.
Uniqueness: this compound is unique in its structure due to the presence of an additional lysine residue at the N-terminal end compared to bradykinin . This structural difference allows this compound to interact with different enzymes and receptors, leading to distinct physiological effects .
Properties
IUPAC Name |
(2S)-2-[[(2S)-2-[[(2S)-1-[(2S)-2-[[(2S)-2-[[2-[[(2S)-1-[(2S)-1-[(2S)-2-[[(2S)-2,6-diaminohexanoyl]amino]-5-(diaminomethylideneamino)pentanoyl]pyrrolidine-2-carbonyl]pyrrolidine-2-carbonyl]amino]acetyl]amino]-3-phenylpropanoyl]amino]-3-hydroxypropanoyl]pyrrolidine-2-carbonyl]amino]-3-phenylpropanoyl]amino]-5-(diaminomethylideneamino)pentanoic acid | |
---|---|---|
Source | PubChem | |
URL | https://pubchem.ncbi.nlm.nih.gov | |
Description | Data deposited in or computed by PubChem | |
InChI |
InChI=1S/C56H85N17O12/c57-24-8-7-18-36(58)46(76)67-37(19-9-25-63-55(59)60)51(81)73-29-13-23-44(73)53(83)72-28-11-21-42(72)49(79)65-32-45(75)66-39(30-34-14-3-1-4-15-34)47(77)70-41(33-74)52(82)71-27-12-22-43(71)50(80)69-40(31-35-16-5-2-6-17-35)48(78)68-38(54(84)85)20-10-26-64-56(61)62/h1-6,14-17,36-44,74H,7-13,18-33,57-58H2,(H,65,79)(H,66,75)(H,67,76)(H,68,78)(H,69,80)(H,70,77)(H,84,85)(H4,59,60,63)(H4,61,62,64)/t36-,37-,38-,39-,40-,41-,42-,43-,44-/m0/s1 | |
Source | PubChem | |
URL | https://pubchem.ncbi.nlm.nih.gov | |
Description | Data deposited in or computed by PubChem | |
InChI Key |
FYSKZKQBTVLYEQ-FSLKYBNLSA-N | |
Source | PubChem | |
URL | https://pubchem.ncbi.nlm.nih.gov | |
Description | Data deposited in or computed by PubChem | |
Canonical SMILES |
C1CC(N(C1)C(=O)C2CCCN2C(=O)C(CCCN=C(N)N)NC(=O)C(CCCCN)N)C(=O)NCC(=O)NC(CC3=CC=CC=C3)C(=O)NC(CO)C(=O)N4CCCC4C(=O)NC(CC5=CC=CC=C5)C(=O)NC(CCCN=C(N)N)C(=O)O | |
Source | PubChem | |
URL | https://pubchem.ncbi.nlm.nih.gov | |
Description | Data deposited in or computed by PubChem | |
Isomeric SMILES |
C1C[C@H](N(C1)C(=O)[C@@H]2CCCN2C(=O)[C@H](CCCN=C(N)N)NC(=O)[C@H](CCCCN)N)C(=O)NCC(=O)N[C@@H](CC3=CC=CC=C3)C(=O)N[C@@H](CO)C(=O)N4CCC[C@H]4C(=O)N[C@@H](CC5=CC=CC=C5)C(=O)N[C@@H](CCCN=C(N)N)C(=O)O | |
Source | PubChem | |
URL | https://pubchem.ncbi.nlm.nih.gov | |
Description | Data deposited in or computed by PubChem | |
Molecular Formula |
C56H85N17O12 | |
Source | PubChem | |
URL | https://pubchem.ncbi.nlm.nih.gov | |
Description | Data deposited in or computed by PubChem | |
DSSTOX Substance ID |
DTXSID40895018 | |
Record name | Kallidin | |
Source | EPA DSSTox | |
URL | https://comptox.epa.gov/dashboard/DTXSID40895018 | |
Description | DSSTox provides a high quality public chemistry resource for supporting improved predictive toxicology. | |
Molecular Weight |
1188.4 g/mol | |
Source | PubChem | |
URL | https://pubchem.ncbi.nlm.nih.gov | |
Description | Data deposited in or computed by PubChem | |
CAS No. |
342-10-9 | |
Record name | Kallidin | |
Source | ChemIDplus | |
URL | https://pubchem.ncbi.nlm.nih.gov/substance/?source=chemidplus&sourceid=0000342109 | |
Description | ChemIDplus is a free, web search system that provides access to the structure and nomenclature authority files used for the identification of chemical substances cited in National Library of Medicine (NLM) databases, including the TOXNET system. | |
Record name | Kallidin | |
Source | EPA DSSTox | |
URL | https://comptox.epa.gov/dashboard/DTXSID40895018 | |
Description | DSSTox provides a high quality public chemistry resource for supporting improved predictive toxicology. | |
Record name | Bradykinin, N2-l-lysyl- | |
Source | European Chemicals Agency (ECHA) | |
URL | https://echa.europa.eu/substance-information/-/substanceinfo/100.005.853 | |
Description | The European Chemicals Agency (ECHA) is an agency of the European Union which is the driving force among regulatory authorities in implementing the EU's groundbreaking chemicals legislation for the benefit of human health and the environment as well as for innovation and competitiveness. | |
Explanation | Use of the information, documents and data from the ECHA website is subject to the terms and conditions of this Legal Notice, and subject to other binding limitations provided for under applicable law, the information, documents and data made available on the ECHA website may be reproduced, distributed and/or used, totally or in part, for non-commercial purposes provided that ECHA is acknowledged as the source: "Source: European Chemicals Agency, http://echa.europa.eu/". Such acknowledgement must be included in each copy of the material. ECHA permits and encourages organisations and individuals to create links to the ECHA website under the following cumulative conditions: Links can only be made to webpages that provide a link to the Legal Notice page. | |
Retrosynthesis Analysis
AI-Powered Synthesis Planning: Our tool employs the Template_relevance Pistachio, Template_relevance Bkms_metabolic, Template_relevance Pistachio_ringbreaker, Template_relevance Reaxys, Template_relevance Reaxys_biocatalysis model, leveraging a vast database of chemical reactions to predict feasible synthetic routes.
One-Step Synthesis Focus: Specifically designed for one-step synthesis, it provides concise and direct routes for your target compounds, streamlining the synthesis process.
Accurate Predictions: Utilizing the extensive PISTACHIO, BKMS_METABOLIC, PISTACHIO_RINGBREAKER, REAXYS, REAXYS_BIOCATALYSIS database, our tool offers high-accuracy predictions, reflecting the latest in chemical research and data.
Strategy Settings
Precursor scoring | Relevance Heuristic |
---|---|
Min. plausibility | 0.01 |
Model | Template_relevance |
Template Set | Pistachio/Bkms_metabolic/Pistachio_ringbreaker/Reaxys/Reaxys_biocatalysis |
Top-N result to add to graph | 6 |
Feasible Synthetic Routes
Disclaimer and Information on In-Vitro Research Products
Please be aware that all articles and product information presented on BenchChem are intended solely for informational purposes. The products available for purchase on BenchChem are specifically designed for in-vitro studies, which are conducted outside of living organisms. In-vitro studies, derived from the Latin term "in glass," involve experiments performed in controlled laboratory settings using cells or tissues. It is important to note that these products are not categorized as medicines or drugs, and they have not received approval from the FDA for the prevention, treatment, or cure of any medical condition, ailment, or disease. We must emphasize that any form of bodily introduction of these products into humans or animals is strictly prohibited by law. It is essential to adhere to these guidelines to ensure compliance with legal and ethical standards in research and experimentation.