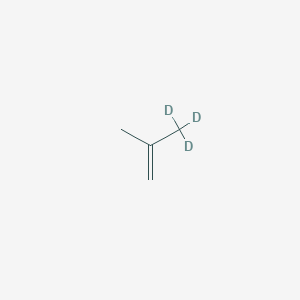
3,3,3-Trideuterio-2-methylprop-1-ene
Overview
Description
This compound is a colorless gas used in various industrial applications, including the production of synthetic rubber, plastics, and fuel additives.
Preparation Methods
Synthetic Routes and Reaction Conditions
The synthesis of 3,3,3-Trideuterio-2-methylprop-1-ene typically involves the deuteration of 2-methylpropene. This process can be achieved through the catalytic exchange of hydrogen atoms with deuterium atoms using deuterium gas (D2) in the presence of a suitable catalyst, such as palladium on carbon (Pd/C). The reaction conditions often include elevated temperatures and pressures to facilitate the exchange process.
Industrial Production Methods
In an industrial setting, the production of this compound may involve continuous flow reactors to ensure efficient deuteration. The use of deuterium gas in large quantities and the recycling of unreacted deuterium are common practices to optimize the yield and reduce costs.
Chemical Reactions Analysis
Types of Reactions
3,3,3-Trideuterio-2-methylprop-1-ene undergoes various chemical reactions, including:
Oxidation: The compound can be oxidized to form corresponding alcohols, aldehydes, or carboxylic acids.
Reduction: Reduction reactions can convert it into saturated hydrocarbons.
Substitution: It can participate in substitution reactions where deuterium atoms are replaced by other functional groups.
Common Reagents and Conditions
Oxidation: Common oxidizing agents include potassium permanganate (KMnO4) and chromium trioxide (CrO3).
Reduction: Catalytic hydrogenation using hydrogen gas (H2) and a catalyst such as palladium on carbon (Pd/C).
Substitution: Halogenation reactions using halogens like chlorine (Cl2) or bromine (Br2) under controlled conditions.
Major Products
Oxidation: Formation of deuterated alcohols, aldehydes, or carboxylic acids.
Reduction: Formation of deuterated alkanes.
Substitution: Formation of deuterated halides.
Scientific Research Applications
3,3,3-Trideuterio-2-methylprop-1-ene is used in various scientific research applications, including:
Chemistry: As a deuterated compound, it is used in nuclear magnetic resonance (NMR) spectroscopy to study reaction mechanisms and molecular structures.
Biology: It serves as a tracer in metabolic studies to understand biochemical pathways.
Medicine: Used in the development of deuterated drugs to improve pharmacokinetic properties.
Industry: Employed in the production of deuterated polymers and materials with unique properties.
Mechanism of Action
The mechanism by which 3,3,3-Trideuterio-2-methylprop-1-ene exerts its effects involves the replacement of hydrogen atoms with deuterium atoms. This isotopic substitution can alter the compound’s physical and chemical properties, such as bond strength and reaction rates. The molecular targets and pathways involved depend on the specific application, such as its role as a tracer in metabolic studies or its use in NMR spectroscopy.
Comparison with Similar Compounds
Similar Compounds
2-Methylpropene (Isobutylene): The non-deuterated form of 3,3,3-Trideuterio-2-methylprop-1-ene.
3,3,3-Trideuterio-2-methylpropane: A fully saturated deuterated derivative.
Deuterated Ethylene: Another deuterated hydrocarbon used in similar applications.
Uniqueness
This compound is unique due to its specific deuteration pattern, which makes it particularly useful in NMR spectroscopy and as a tracer in metabolic studies. Its deuterium atoms provide distinct advantages in studying reaction mechanisms and improving the stability and efficacy of deuterated drugs.
Properties
IUPAC Name |
3,3,3-trideuterio-2-methylprop-1-ene | |
---|---|---|
Source | PubChem | |
URL | https://pubchem.ncbi.nlm.nih.gov | |
Description | Data deposited in or computed by PubChem | |
InChI |
InChI=1S/C4H8/c1-4(2)3/h1H2,2-3H3/i2D3 | |
Source | PubChem | |
URL | https://pubchem.ncbi.nlm.nih.gov | |
Description | Data deposited in or computed by PubChem | |
InChI Key |
VQTUBCCKSQIDNK-BMSJAHLVSA-N | |
Source | PubChem | |
URL | https://pubchem.ncbi.nlm.nih.gov | |
Description | Data deposited in or computed by PubChem | |
Canonical SMILES |
CC(=C)C | |
Source | PubChem | |
URL | https://pubchem.ncbi.nlm.nih.gov | |
Description | Data deposited in or computed by PubChem | |
Isomeric SMILES |
[2H]C([2H])([2H])C(=C)C | |
Source | PubChem | |
URL | https://pubchem.ncbi.nlm.nih.gov | |
Description | Data deposited in or computed by PubChem | |
Molecular Formula |
C4H8 | |
Source | PubChem | |
URL | https://pubchem.ncbi.nlm.nih.gov | |
Description | Data deposited in or computed by PubChem | |
Molecular Weight |
59.12 g/mol | |
Source | PubChem | |
URL | https://pubchem.ncbi.nlm.nih.gov | |
Description | Data deposited in or computed by PubChem | |
Synthesis routes and methods I
Procedure details
Synthesis routes and methods II
Procedure details
Synthesis routes and methods III
Procedure details
Synthesis routes and methods IV
Procedure details
Synthesis routes and methods V
Procedure details
Retrosynthesis Analysis
AI-Powered Synthesis Planning: Our tool employs the Template_relevance Pistachio, Template_relevance Bkms_metabolic, Template_relevance Pistachio_ringbreaker, Template_relevance Reaxys, Template_relevance Reaxys_biocatalysis model, leveraging a vast database of chemical reactions to predict feasible synthetic routes.
One-Step Synthesis Focus: Specifically designed for one-step synthesis, it provides concise and direct routes for your target compounds, streamlining the synthesis process.
Accurate Predictions: Utilizing the extensive PISTACHIO, BKMS_METABOLIC, PISTACHIO_RINGBREAKER, REAXYS, REAXYS_BIOCATALYSIS database, our tool offers high-accuracy predictions, reflecting the latest in chemical research and data.
Strategy Settings
Precursor scoring | Relevance Heuristic |
---|---|
Min. plausibility | 0.01 |
Model | Template_relevance |
Template Set | Pistachio/Bkms_metabolic/Pistachio_ringbreaker/Reaxys/Reaxys_biocatalysis |
Top-N result to add to graph | 6 |
Feasible Synthetic Routes
Disclaimer and Information on In-Vitro Research Products
Please be aware that all articles and product information presented on BenchChem are intended solely for informational purposes. The products available for purchase on BenchChem are specifically designed for in-vitro studies, which are conducted outside of living organisms. In-vitro studies, derived from the Latin term "in glass," involve experiments performed in controlled laboratory settings using cells or tissues. It is important to note that these products are not categorized as medicines or drugs, and they have not received approval from the FDA for the prevention, treatment, or cure of any medical condition, ailment, or disease. We must emphasize that any form of bodily introduction of these products into humans or animals is strictly prohibited by law. It is essential to adhere to these guidelines to ensure compliance with legal and ethical standards in research and experimentation.