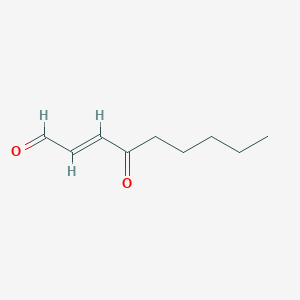
4-Oxo-2-nonenal
Overview
Description
4-Oxo-2-Nonenal is a lipid peroxidation product that can structurally alter proteins and induce α-synuclein oligomers . It is a highly reactive aldehyde formed during the oxidative degradation of polyunsaturated fatty acids. This compound is known for its role in various biological processes and its potential implications in diseases such as Parkinson’s disease .
Mechanism of Action
Target of Action
4-Oxo-2-nonenal (4-ONE), a lipid peroxidation product, primarily targets proteins, specifically histidine and lysine residues . It actively modifies these residues, leading to protein cross-linking . Another major target of 4-ONE is α-Synuclein (α-syn), a protein associated with the pathogenesis of Parkinson’s disease and other synucleinopathies . 4-ONE can induce aggregation of α-syn and phosphorylated α-syn .
Mode of Action
4-ONE interacts with its targets by modifying nucleophiles and transducing redox signaling through its reactions with proteins . It forms ketoamide and Schiff-base adducts to lysine, Michael adducts to cysteine, and a novel pyrrole adduct to cysteine . These modifications co-localize and exhibit crosstalk with many histone marks and redox-sensitive sites .
Biochemical Pathways
The primary biochemical pathway affected by 4-ONE involves lipid peroxidation. 4-ONE is derived from lipid peroxidation, a process that modifies nucleophiles and transduces redox signaling . The polyunsaturated fatty acyl chains found in biological membranes and lipoproteins are particularly susceptible to reactive oxygen species, leading to free radical chain autoxidation and the formation of a variety of unsaturated lipid hydroperoxides .
Result of Action
The action of 4-ONE results in distinct biochemical, morphological, and functional properties. It promotes the formation of α-synuclein oligomers , which are associated with the pathogenesis of Parkinson’s disease and other synucleinopathies . Furthermore, 4-ONE-induced aggregates were found to be cytotoxic when added exogenously to SH-SY5Y cells .
Action Environment
The action of 4-ONE can be influenced by environmental factors such as the presence of reactive oxygen species, which can lead to lipid peroxidation and the formation of 4-ONE . Additionally, the agitation can induce the aggregation of α-syn and phosphorylated α-syn . The stability and compactness of 4-ONE-induced aggregates were found to be greater than those of agitation-induced aggregates .
Biochemical Analysis
Biochemical Properties
4-Oxo-2-nonenal can induce aggregation of α-synuclein and phosphorylated α-synuclein . It forms four types of modifications in cells, including ketoamide and Schiff-base adducts to lysine, Michael adducts to cysteine, and a novel pyrrole adduct to cysteine .
Cellular Effects
This compound has been found to cause damage to mitochondrial structure and impairment of myoglobin’s redox stability . It also influences cell function by causing cytotoxicity when added exogenously to SH-SY5Y cells with increasing incubation times .
Molecular Mechanism
The molecular mechanism of this compound involves its reactions with proteins. It forms adducts with proteins, which can affect their function . For example, it forms adducts with α-synuclein, which can lead to the formation of aggregates .
Temporal Effects in Laboratory Settings
Over time, this compound can cause changes in cellular function. For example, it has been found to cause cytotoxicity in SH-SY5Y cells over time .
Metabolic Pathways
This compound is involved in lipid peroxidation, a metabolic pathway that leads to the formation of reactive oxygen species . It can react with proteins to form adducts, which can affect their function .
Preparation Methods
Synthetic Routes and Reaction Conditions: 4-Oxo-2-Nonenal can be synthesized through the oxidation of 2-nonenal. The reaction typically involves the use of oxidizing agents such as potassium permanganate or chromium trioxide under controlled conditions to achieve the desired product .
Industrial Production Methods: In an industrial setting, this compound is produced through lipid peroxidation processes. This involves the oxidation of polyunsaturated fatty acids, such as arachidonic acid and linoleic acid, in the presence of reactive oxygen species . The process is carefully monitored to ensure the purity and yield of the compound.
Chemical Reactions Analysis
Types of Reactions: 4-Oxo-2-Nonenal undergoes various chemical reactions, including:
Oxidation: It can be further oxidized to form carboxylic acids.
Reduction: It can be reduced to form alcohols.
Substitution: It can react with nucleophiles such as amines and thiols to form adducts.
Common Reagents and Conditions:
Oxidation: Potassium permanganate, chromium trioxide.
Reduction: Sodium borohydride, lithium aluminum hydride.
Substitution: Amines, thiols, under mild conditions.
Major Products Formed:
Oxidation: Carboxylic acids.
Reduction: Alcohols.
Substitution: Adducts with amines and thiols.
Scientific Research Applications
4-Oxo-2-Nonenal has a wide range of applications in scientific research:
Comparison with Similar Compounds
4-Hydroxy-2-Nonenal: Another lipid peroxidation product with similar reactivity and biological effects.
Malondialdehyde: A smaller aldehyde formed during lipid peroxidation, known for its role in oxidative stress.
Uniqueness of 4-Oxo-2-Nonenal: this compound is unique due to its ability to form stable adducts with proteins and DNA, leading to significant structural and functional changes. Its role in protein aggregation and potential involvement in neurodegenerative diseases sets it apart from other lipid peroxidation products .
Properties
IUPAC Name |
(E)-4-oxonon-2-enal | |
---|---|---|
Source | PubChem | |
URL | https://pubchem.ncbi.nlm.nih.gov | |
Description | Data deposited in or computed by PubChem | |
InChI |
InChI=1S/C9H14O2/c1-2-3-4-6-9(11)7-5-8-10/h5,7-8H,2-4,6H2,1H3/b7-5+ | |
Source | PubChem | |
URL | https://pubchem.ncbi.nlm.nih.gov | |
Description | Data deposited in or computed by PubChem | |
InChI Key |
SEPPVOUBHWNCAW-FNORWQNLSA-N | |
Source | PubChem | |
URL | https://pubchem.ncbi.nlm.nih.gov | |
Description | Data deposited in or computed by PubChem | |
Canonical SMILES |
CCCCCC(=O)C=CC=O | |
Source | PubChem | |
URL | https://pubchem.ncbi.nlm.nih.gov | |
Description | Data deposited in or computed by PubChem | |
Isomeric SMILES |
CCCCCC(=O)/C=C/C=O | |
Source | PubChem | |
URL | https://pubchem.ncbi.nlm.nih.gov | |
Description | Data deposited in or computed by PubChem | |
Molecular Formula |
C9H14O2 | |
Source | PubChem | |
URL | https://pubchem.ncbi.nlm.nih.gov | |
Description | Data deposited in or computed by PubChem | |
DSSTOX Substance ID |
DTXSID701032845 | |
Record name | 4-Oxo-2-nonenal | |
Source | EPA DSSTox | |
URL | https://comptox.epa.gov/dashboard/DTXSID701032845 | |
Description | DSSTox provides a high quality public chemistry resource for supporting improved predictive toxicology. | |
Molecular Weight |
154.21 g/mol | |
Source | PubChem | |
URL | https://pubchem.ncbi.nlm.nih.gov | |
Description | Data deposited in or computed by PubChem | |
CAS No. |
103560-62-9 | |
Record name | 4-Oxo-2-(E)-nonenal | |
Source | CAS Common Chemistry | |
URL | https://commonchemistry.cas.org/detail?cas_rn=103560-62-9 | |
Description | CAS Common Chemistry is an open community resource for accessing chemical information. Nearly 500,000 chemical substances from CAS REGISTRY cover areas of community interest, including common and frequently regulated chemicals, and those relevant to high school and undergraduate chemistry classes. This chemical information, curated by our expert scientists, is provided in alignment with our mission as a division of the American Chemical Society. | |
Explanation | The data from CAS Common Chemistry is provided under a CC-BY-NC 4.0 license, unless otherwise stated. | |
Record name | 4-Oxo-2-nonenal | |
Source | ChemIDplus | |
URL | https://pubchem.ncbi.nlm.nih.gov/substance/?source=chemidplus&sourceid=0103560629 | |
Description | ChemIDplus is a free, web search system that provides access to the structure and nomenclature authority files used for the identification of chemical substances cited in National Library of Medicine (NLM) databases, including the TOXNET system. | |
Record name | 4-Oxo-2-nonenal | |
Source | EPA DSSTox | |
URL | https://comptox.epa.gov/dashboard/DTXSID701032845 | |
Description | DSSTox provides a high quality public chemistry resource for supporting improved predictive toxicology. | |
Retrosynthesis Analysis
AI-Powered Synthesis Planning: Our tool employs the Template_relevance Pistachio, Template_relevance Bkms_metabolic, Template_relevance Pistachio_ringbreaker, Template_relevance Reaxys, Template_relevance Reaxys_biocatalysis model, leveraging a vast database of chemical reactions to predict feasible synthetic routes.
One-Step Synthesis Focus: Specifically designed for one-step synthesis, it provides concise and direct routes for your target compounds, streamlining the synthesis process.
Accurate Predictions: Utilizing the extensive PISTACHIO, BKMS_METABOLIC, PISTACHIO_RINGBREAKER, REAXYS, REAXYS_BIOCATALYSIS database, our tool offers high-accuracy predictions, reflecting the latest in chemical research and data.
Strategy Settings
Precursor scoring | Relevance Heuristic |
---|---|
Min. plausibility | 0.01 |
Model | Template_relevance |
Template Set | Pistachio/Bkms_metabolic/Pistachio_ringbreaker/Reaxys/Reaxys_biocatalysis |
Top-N result to add to graph | 6 |
Feasible Synthetic Routes
Disclaimer and Information on In-Vitro Research Products
Please be aware that all articles and product information presented on BenchChem are intended solely for informational purposes. The products available for purchase on BenchChem are specifically designed for in-vitro studies, which are conducted outside of living organisms. In-vitro studies, derived from the Latin term "in glass," involve experiments performed in controlled laboratory settings using cells or tissues. It is important to note that these products are not categorized as medicines or drugs, and they have not received approval from the FDA for the prevention, treatment, or cure of any medical condition, ailment, or disease. We must emphasize that any form of bodily introduction of these products into humans or animals is strictly prohibited by law. It is essential to adhere to these guidelines to ensure compliance with legal and ethical standards in research and experimentation.