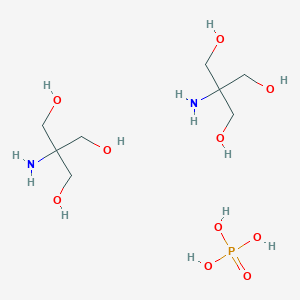
Tris phosphate dibasic
Overview
Description
Tris phosphate dibasic, also known as trisodium phosphate, is an inorganic compound with the chemical formula Na₃PO₄. It is a white, granular or crystalline solid that is highly soluble in water, producing an alkaline solution. This compound is commonly used in various industrial and scientific applications due to its buffering and cleaning properties .
Scientific Research Applications
Tris phosphate dibasic is widely used in scientific research due to its buffering capacity and chemical properties. Some of its applications include:
Chemistry: Used as a reagent in various chemical reactions and as a buffering agent in analytical chemistry.
Biology: Employed in the preparation of buffer solutions for biological experiments, such as phosphate-buffered saline (PBS).
Medicine: Utilized in pharmaceutical formulations and as a laxative in medical treatments.
Industry: Applied in water treatment, cleaning agents, and as a food additive.
Safety and Hazards
Mechanism of Action
Target of Action
Tris phosphate dibasic, also known as Trisodium phosphate (TSP), is an inorganic compound with the chemical formula Na3PO4 . It is primarily used as a cleaning agent, builder, lubricant, food additive, stain remover, and degreaser . The primary targets of TSP are the molecules and structures it interacts with in these applications, such as dirt and grease molecules in cleaning applications, or various food molecules when used as a food additive.
Mode of Action
TSP is highly soluble in water, producing an alkaline solution . This alkalinity allows it to effectively break down greasy and dirty surfaces in cleaning applications In food applications, it can serve as a pH adjuster or buffering agent.
Preparation Methods
Synthetic Routes and Reaction Conditions
Tris phosphate dibasic is typically synthesized through the neutralization of phosphoric acid (H₃PO₄) with sodium carbonate (Na₂CO₃). The reaction produces disodium hydrogen phosphate (Na₂HPO₄), which is then reacted with sodium hydroxide (NaOH) to form trisodium phosphate and water: [ \text{H}_3\text{PO}_4 + 2\text{Na}_2\text{CO}_3 \rightarrow 2\text{Na}_2\text{HPO}_4 + \text{CO}_2 + \text{H}_2\text{O} ] [ \text{Na}_2\text{HPO}_4 + \text{NaOH} \rightarrow \text{Na}_3\text{PO}_4 + \text{H}_2\text{O} ]
Industrial Production Methods
In industrial settings, this compound is produced in large quantities using similar neutralization reactions. The process involves careful control of reaction conditions, such as temperature and pH, to ensure high purity and yield of the final product .
Chemical Reactions Analysis
Types of Reactions
Tris phosphate dibasic undergoes various chemical reactions, including:
Hydrolysis: In aqueous solutions, it dissociates into sodium ions (Na⁺) and phosphate ions (PO₄³⁻).
Complexation: It can form complexes with metal ions, which is useful in water treatment processes.
Neutralization: It reacts with acids to form corresponding salts and water.
Common Reagents and Conditions
Acids: Reacts with hydrochloric acid (HCl) to form sodium chloride (NaCl) and phosphoric acid.
Metal Ions: Forms complexes with calcium (Ca²⁺), magnesium (Mg²⁺), and other metal ions.
Major Products Formed
Sodium chloride (NaCl): Formed during neutralization reactions with hydrochloric acid.
Metal complexes: Formed during reactions with metal ions.
Comparison with Similar Compounds
Tris phosphate dibasic can be compared with other similar compounds, such as:
Monosodium phosphate (NaH₂PO₄): Used as a buffering agent but has a lower pH range compared to this compound.
Disodium phosphate (Na₂HPO₄): Also used as a buffering agent with a pH range between monosodium phosphate and this compound.
Tripotassium phosphate (K₃PO₄): Similar buffering properties but uses potassium ions instead of sodium ions.
This compound is unique due to its high solubility in water and strong buffering capacity, making it suitable for a wide range of applications in various fields.
Properties
IUPAC Name |
2-amino-2-(hydroxymethyl)propane-1,3-diol;phosphoric acid | |
---|---|---|
Source | PubChem | |
URL | https://pubchem.ncbi.nlm.nih.gov | |
Description | Data deposited in or computed by PubChem | |
InChI |
InChI=1S/2C4H11NO3.H3O4P/c2*5-4(1-6,2-7)3-8;1-5(2,3)4/h2*6-8H,1-3,5H2;(H3,1,2,3,4) | |
Source | PubChem | |
URL | https://pubchem.ncbi.nlm.nih.gov | |
Description | Data deposited in or computed by PubChem | |
InChI Key |
MYASHAYBKIUKNJ-UHFFFAOYSA-N | |
Source | PubChem | |
URL | https://pubchem.ncbi.nlm.nih.gov | |
Description | Data deposited in or computed by PubChem | |
Canonical SMILES |
C(C(CO)(CO)N)O.C(C(CO)(CO)N)O.OP(=O)(O)O | |
Source | PubChem | |
URL | https://pubchem.ncbi.nlm.nih.gov | |
Description | Data deposited in or computed by PubChem | |
Molecular Formula |
C8H25N2O10P | |
Source | PubChem | |
URL | https://pubchem.ncbi.nlm.nih.gov | |
Description | Data deposited in or computed by PubChem | |
DSSTOX Substance ID |
DTXSID30585274 | |
Record name | Phosphoric acid--2-amino-2-(hydroxymethyl)propane-1,3-diol (1/2) | |
Source | EPA DSSTox | |
URL | https://comptox.epa.gov/dashboard/DTXSID30585274 | |
Description | DSSTox provides a high quality public chemistry resource for supporting improved predictive toxicology. | |
Molecular Weight |
340.27 g/mol | |
Source | PubChem | |
URL | https://pubchem.ncbi.nlm.nih.gov | |
Description | Data deposited in or computed by PubChem | |
CAS No. |
108321-11-5 | |
Record name | Phosphoric acid--2-amino-2-(hydroxymethyl)propane-1,3-diol (1/2) | |
Source | EPA DSSTox | |
URL | https://comptox.epa.gov/dashboard/DTXSID30585274 | |
Description | DSSTox provides a high quality public chemistry resource for supporting improved predictive toxicology. | |
Retrosynthesis Analysis
AI-Powered Synthesis Planning: Our tool employs the Template_relevance Pistachio, Template_relevance Bkms_metabolic, Template_relevance Pistachio_ringbreaker, Template_relevance Reaxys, Template_relevance Reaxys_biocatalysis model, leveraging a vast database of chemical reactions to predict feasible synthetic routes.
One-Step Synthesis Focus: Specifically designed for one-step synthesis, it provides concise and direct routes for your target compounds, streamlining the synthesis process.
Accurate Predictions: Utilizing the extensive PISTACHIO, BKMS_METABOLIC, PISTACHIO_RINGBREAKER, REAXYS, REAXYS_BIOCATALYSIS database, our tool offers high-accuracy predictions, reflecting the latest in chemical research and data.
Strategy Settings
Precursor scoring | Relevance Heuristic |
---|---|
Min. plausibility | 0.01 |
Model | Template_relevance |
Template Set | Pistachio/Bkms_metabolic/Pistachio_ringbreaker/Reaxys/Reaxys_biocatalysis |
Top-N result to add to graph | 6 |
Feasible Synthetic Routes
Disclaimer and Information on In-Vitro Research Products
Please be aware that all articles and product information presented on BenchChem are intended solely for informational purposes. The products available for purchase on BenchChem are specifically designed for in-vitro studies, which are conducted outside of living organisms. In-vitro studies, derived from the Latin term "in glass," involve experiments performed in controlled laboratory settings using cells or tissues. It is important to note that these products are not categorized as medicines or drugs, and they have not received approval from the FDA for the prevention, treatment, or cure of any medical condition, ailment, or disease. We must emphasize that any form of bodily introduction of these products into humans or animals is strictly prohibited by law. It is essential to adhere to these guidelines to ensure compliance with legal and ethical standards in research and experimentation.