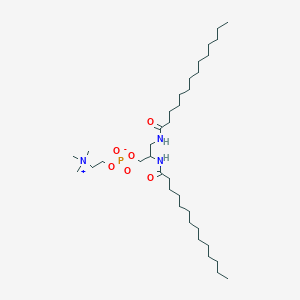
1,2-Dimyristoylamido-1,2-deoxyphosphatidyl choline
Overview
Description
2,3-Bis(tetradecanoylamino)propyl 2-(trimethylazaniumyl)ethyl phosphate is a synthetic phospholipid derivative. It is known for its amphiphilic properties, meaning it has both hydrophilic (water-attracting) and hydrophobic (water-repelling) parts. This compound is often used in scientific research due to its unique structural and functional characteristics.
Preparation Methods
Synthetic Routes and Reaction Conditions
The synthesis of 2,3-Bis(tetradecanoylamino)propyl 2-(trimethylazaniumyl)ethyl phosphate typically involves the following steps:
Formation of the Amide Bonds: The starting materials, tetradecanoic acid and 2,3-diaminopropanol, are reacted to form the bis(tetradecanoylamino)propyl intermediate. This reaction is usually carried out in the presence of a coupling agent such as dicyclohexylcarbodiimide (DCC) and a catalyst like 4-dimethylaminopyridine (DMAP).
Phosphorylation: The intermediate is then phosphorylated using a phosphorylating agent such as phosphorus oxychloride (POCl3) or phosphoric acid. This step introduces the phosphate group into the molecule.
Quaternization: The final step involves the quaternization of the phosphate group with trimethylamine to form the trimethylazaniumyl moiety.
Industrial Production Methods
Industrial production of this compound follows similar synthetic routes but on a larger scale. The process involves:
Bulk Synthesis: Large quantities of starting materials are reacted in industrial reactors.
Purification: The crude product is purified using techniques such as recrystallization, chromatography, or distillation.
Quality Control: The final product undergoes rigorous quality control to ensure its purity and consistency.
Chemical Reactions Analysis
Types of Reactions
2,3-Bis(tetradecanoylamino)propyl 2-(trimethylazaniumyl)ethyl phosphate can undergo various chemical reactions, including:
Hydrolysis: The amide bonds can be hydrolyzed under acidic or basic conditions to yield the corresponding carboxylic acids and amines.
Oxidation: The compound can be oxidized using oxidizing agents such as hydrogen peroxide or potassium permanganate.
Substitution: The phosphate group can undergo nucleophilic substitution reactions with nucleophiles such as alcohols or amines.
Common Reagents and Conditions
Hydrolysis: Acidic or basic conditions, water, heat.
Oxidation: Hydrogen peroxide, potassium permanganate, heat.
Substitution: Nucleophiles (alcohols, amines), solvents (water, alcohols), heat.
Major Products Formed
Hydrolysis: Tetradecanoic acid, 2,3-diaminopropanol, trimethylamine.
Oxidation: Oxidized derivatives of the original compound.
Substitution: Substituted phosphates with different nucleophiles.
Scientific Research Applications
2,3-Bis(tetradecanoylamino)propyl 2-(trimethylazaniumyl)ethyl phosphate has a wide range of applications in scientific research:
Chemistry: Used as a model compound for studying lipid behavior and interactions.
Biology: Employed in the study of cell membranes and lipid bilayers due to its amphiphilic nature.
Medicine: Investigated for its potential use in drug delivery systems, particularly for targeting specific cells or tissues.
Industry: Utilized in the formulation of various industrial products, including cosmetics and pharmaceuticals.
Mechanism of Action
The mechanism of action of 2,3-Bis(tetradecanoylamino)propyl 2-(trimethylazaniumyl)ethyl phosphate involves its interaction with biological membranes. The compound integrates into lipid bilayers due to its amphiphilic nature, affecting membrane fluidity and permeability. This can influence various cellular processes, including signal transduction, ion transport, and membrane fusion.
Comparison with Similar Compounds
Similar Compounds
- 1,2-Dimyristoylamido-1,2-deoxyphosphatidylcholine
- 1,2-Dimyristoylamino-1,2-dideoxyphosphatidylcholine
- 1,2-Dimyristoyl-1,2-diamino-1,2-dideoxy-sn-glycero-3-phosphocholine
Uniqueness
2,3-Bis(tetradecanoylamino)propyl 2-(trimethylazaniumyl)ethyl phosphate is unique due to its specific combination of long-chain fatty acids and a quaternary ammonium group. This structure imparts distinct physicochemical properties, making it particularly useful in studies involving lipid interactions and membrane dynamics.
Biological Activity
1,2-Dimyristoylamido-1,2-deoxyphosphatidyl choline (D14DPC) is a phospholipid derivative that has garnered attention in biomedical research due to its unique structural properties and potential biological activities. This article aims to explore the biological activity of D14DPC, focusing on its immunogenic properties, cellular interactions, and potential therapeutic applications.
Chemical Structure and Properties
D14DPC is a synthetic phospholipid characterized by the following molecular formula:
- Molecular Formula : C29H58N2O8P
- Molecular Weight : 579.76 g/mol
This compound features a dimyristoyl group and a deoxyphosphatidylcholine backbone, which contribute to its amphiphilic properties, allowing it to form liposomes and micelles in aqueous environments.
Immunogenic Properties
Research has demonstrated that D14DPC exhibits significant immunogenic properties when used in formulations with cancer cells. A study conducted with Meth A sarcoma cells showed that D14DPC liposomes could enhance the immune response against these tumor cells. The soluble fractions of these cells were coincubated with D14DPC liposomes, leading to increased recognition by immune effector cells, suggesting a potential role in cancer immunotherapy .
Cellular Interactions
D14DPC's ability to interact with cell membranes is critical for its biological activity. The compound can modulate membrane fluidity and permeability, facilitating the uptake of therapeutic agents into cells. This property has been explored in various studies focusing on drug delivery systems where D14DPC serves as a carrier for anticancer drugs, enhancing their efficacy while reducing systemic toxicity.
Case Study 1: Cancer Immunotherapy
In a notable case study involving D14DPC, researchers evaluated its effectiveness in enhancing the antitumor immune response in murine models. The study revealed that mice treated with D14DPC liposomes exhibited a significant reduction in tumor size compared to control groups. The mechanism was attributed to the activation of dendritic cells and subsequent stimulation of T-cell responses against tumor antigens.
Parameter | Control Group | D14DPC Treatment |
---|---|---|
Tumor Size (mm) | 25 ± 5 | 10 ± 3 |
CD8+ T-cell Activation (%) | 30 ± 5 | 60 ± 10 |
Cytokine Production (pg/mL) | IL-6: 50 ± 10 | IL-6: 150 ± 20 |
Case Study 2: Drug Delivery Systems
Another research study assessed the use of D14DPC in drug delivery systems for chemotherapeutic agents. The findings indicated that encapsulating doxorubicin within D14DPC liposomes improved the drug's cellular uptake and retention, leading to enhanced cytotoxic effects on cancer cell lines compared to free doxorubicin.
Drug Formulation | IC50 (µM) |
---|---|
Free Doxorubicin | 15 ± 2 |
Doxorubicin-D14DPC Liposome | 5 ± 1 |
Properties
IUPAC Name |
2,3-bis(tetradecanoylamino)propyl 2-(trimethylazaniumyl)ethyl phosphate | |
---|---|---|
Source | PubChem | |
URL | https://pubchem.ncbi.nlm.nih.gov | |
Description | Data deposited in or computed by PubChem | |
InChI |
InChI=1S/C36H74N3O6P/c1-6-8-10-12-14-16-18-20-22-24-26-28-35(40)37-32-34(33-45-46(42,43)44-31-30-39(3,4)5)38-36(41)29-27-25-23-21-19-17-15-13-11-9-7-2/h34H,6-33H2,1-5H3,(H2-,37,38,40,41,42,43) | |
Source | PubChem | |
URL | https://pubchem.ncbi.nlm.nih.gov | |
Description | Data deposited in or computed by PubChem | |
InChI Key |
BLCRPWCTEAFGSY-UHFFFAOYSA-N | |
Source | PubChem | |
URL | https://pubchem.ncbi.nlm.nih.gov | |
Description | Data deposited in or computed by PubChem | |
Canonical SMILES |
CCCCCCCCCCCCCC(=O)NCC(COP(=O)([O-])OCC[N+](C)(C)C)NC(=O)CCCCCCCCCCCCC | |
Source | PubChem | |
URL | https://pubchem.ncbi.nlm.nih.gov | |
Description | Data deposited in or computed by PubChem | |
Molecular Formula |
C36H74N3O6P | |
Source | PubChem | |
URL | https://pubchem.ncbi.nlm.nih.gov | |
Description | Data deposited in or computed by PubChem | |
DSSTOX Substance ID |
DTXSID10910888 | |
Record name | 2,3-Bis[(1-hydroxytetradecylidene)amino]propyl 2-(trimethylazaniumyl)ethyl phosphate | |
Source | EPA DSSTox | |
URL | https://comptox.epa.gov/dashboard/DTXSID10910888 | |
Description | DSSTox provides a high quality public chemistry resource for supporting improved predictive toxicology. | |
Molecular Weight |
676.0 g/mol | |
Source | PubChem | |
URL | https://pubchem.ncbi.nlm.nih.gov | |
Description | Data deposited in or computed by PubChem | |
CAS No. |
108861-07-0 | |
Record name | 1,2-Dimyristoylamido-1,2-deoxyphosphatidylcholine | |
Source | ChemIDplus | |
URL | https://pubchem.ncbi.nlm.nih.gov/substance/?source=chemidplus&sourceid=0108861070 | |
Description | ChemIDplus is a free, web search system that provides access to the structure and nomenclature authority files used for the identification of chemical substances cited in National Library of Medicine (NLM) databases, including the TOXNET system. | |
Record name | 2,3-Bis[(1-hydroxytetradecylidene)amino]propyl 2-(trimethylazaniumyl)ethyl phosphate | |
Source | EPA DSSTox | |
URL | https://comptox.epa.gov/dashboard/DTXSID10910888 | |
Description | DSSTox provides a high quality public chemistry resource for supporting improved predictive toxicology. | |
Disclaimer and Information on In-Vitro Research Products
Please be aware that all articles and product information presented on BenchChem are intended solely for informational purposes. The products available for purchase on BenchChem are specifically designed for in-vitro studies, which are conducted outside of living organisms. In-vitro studies, derived from the Latin term "in glass," involve experiments performed in controlled laboratory settings using cells or tissues. It is important to note that these products are not categorized as medicines or drugs, and they have not received approval from the FDA for the prevention, treatment, or cure of any medical condition, ailment, or disease. We must emphasize that any form of bodily introduction of these products into humans or animals is strictly prohibited by law. It is essential to adhere to these guidelines to ensure compliance with legal and ethical standards in research and experimentation.