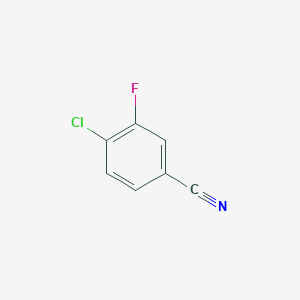
4-Chloro-3-fluorobenzonitrile
Overview
Description
4-Chloro-3-fluorobenzonitrile is an organic compound with the molecular formula C₇H₃ClFN and a molecular weight of 155.56 g/mol . It is a white to orange crystalline powder that is soluble in methanol . This compound is primarily used as an intermediate in the synthesis of other fluorobenzonitriles and has applications in various fields such as pharmaceuticals and agrochemicals .
Mechanism of Action
Target of Action
4-Chloro-3-fluorobenzonitrile is a key intermediate in the synthesis of various fluorobenzonitriles . .
Mode of Action
It’s primarily used as a chemical intermediate, suggesting its role is more likely in the synthesis of other compounds rather than having a direct biological effect .
Biochemical Pathways
As a chemical intermediate, this compound is involved in the synthesis of 3,4-Difluorobenzonitrile
Result of Action
As a chemical intermediate, its primary role is likely in the synthesis of other compounds rather than having a direct biological effect .
Preparation Methods
Synthetic Routes and Reaction Conditions: 4-Chloro-3-fluorobenzonitrile can be synthesized through a halogen exchange reaction. One common method involves the reaction of 3,4-dichlorobenzonitrile with an alkali metal fluoride, such as potassium fluoride, in the presence of a phase transfer catalyst . The reaction typically occurs in an aprotic dipolar solvent like dimethyl sulfoxide at elevated temperatures .
Industrial Production Methods: Industrial production of this compound often employs similar halogen exchange reactions but on a larger scale. The use of continuous flow reactors and optimized reaction conditions can enhance yield and purity while reducing reaction times .
Chemical Reactions Analysis
Types of Reactions: 4-Chloro-3-fluorobenzonitrile undergoes various chemical reactions, including:
Substitution Reactions: It can participate in nucleophilic aromatic substitution reactions where the chlorine or fluorine atom is replaced by other nucleophiles.
Oxidation and Reduction: The compound can be oxidized or reduced under specific conditions to form different derivatives.
Common Reagents and Conditions:
Nucleophilic Substitution: Common reagents include sodium methoxide or potassium tert-butoxide in solvents like dimethyl sulfoxide.
Oxidation: Reagents such as potassium permanganate or chromium trioxide can be used.
Reduction: Reducing agents like lithium aluminum hydride or sodium borohydride are commonly employed.
Major Products Formed:
Substitution Products: Depending on the nucleophile used, various substituted benzonitriles can be formed.
Oxidation Products: Oxidation can yield compounds like benzoic acids or benzamides.
Reduction Products: Reduction typically produces amines or other reduced derivatives.
Scientific Research Applications
4-Chloro-3-fluorobenzonitrile has a wide range of applications in scientific research:
Chemistry: It is used as a building block in the synthesis of more complex organic molecules.
Biology: The compound is utilized in the development of bioactive molecules and pharmaceuticals.
Medicine: It serves as an intermediate in the synthesis of drugs and therapeutic agents.
Industry: The compound is employed in the production of agrochemicals, dyes, and other industrial chemicals.
Comparison with Similar Compounds
- 2-Chloro-3-fluorobenzonitrile
- 3-Chloro-2-fluorobenzonitrile
- 2-Chloro-4-fluorobenzonitrile
- 3-Fluoro-4-chlorotoluene
- 4-Chlorobenzonitrile
- 4-Chloro-2,5-difluorobenzonitrile
Comparison: 4-Chloro-3-fluorobenzonitrile is unique due to its specific substitution pattern on the benzene ring, which imparts distinct reactivity and properties. Compared to its analogs, it offers a balance of reactivity and stability, making it a versatile intermediate in organic synthesis .
Properties
IUPAC Name |
4-chloro-3-fluorobenzonitrile | |
---|---|---|
Source | PubChem | |
URL | https://pubchem.ncbi.nlm.nih.gov | |
Description | Data deposited in or computed by PubChem | |
InChI |
InChI=1S/C7H3ClFN/c8-6-2-1-5(4-10)3-7(6)9/h1-3H | |
Source | PubChem | |
URL | https://pubchem.ncbi.nlm.nih.gov | |
Description | Data deposited in or computed by PubChem | |
InChI Key |
GWZQVECNESCSKR-UHFFFAOYSA-N | |
Source | PubChem | |
URL | https://pubchem.ncbi.nlm.nih.gov | |
Description | Data deposited in or computed by PubChem | |
Canonical SMILES |
C1=CC(=C(C=C1C#N)F)Cl | |
Source | PubChem | |
URL | https://pubchem.ncbi.nlm.nih.gov | |
Description | Data deposited in or computed by PubChem | |
Molecular Formula |
C7H3ClFN | |
Source | PubChem | |
URL | https://pubchem.ncbi.nlm.nih.gov | |
Description | Data deposited in or computed by PubChem | |
DSSTOX Substance ID |
DTXSID80371427 | |
Record name | 4-Chloro-3-fluorobenzonitrile | |
Source | EPA DSSTox | |
URL | https://comptox.epa.gov/dashboard/DTXSID80371427 | |
Description | DSSTox provides a high quality public chemistry resource for supporting improved predictive toxicology. | |
Molecular Weight |
155.55 g/mol | |
Source | PubChem | |
URL | https://pubchem.ncbi.nlm.nih.gov | |
Description | Data deposited in or computed by PubChem | |
CAS No. |
110888-15-8 | |
Record name | 4-Chloro-3-fluorobenzonitrile | |
Source | EPA DSSTox | |
URL | https://comptox.epa.gov/dashboard/DTXSID80371427 | |
Description | DSSTox provides a high quality public chemistry resource for supporting improved predictive toxicology. | |
Record name | 4-Chloro-3-fluorobenzonitrile | |
Source | European Chemicals Agency (ECHA) | |
URL | https://echa.europa.eu/information-on-chemicals | |
Description | The European Chemicals Agency (ECHA) is an agency of the European Union which is the driving force among regulatory authorities in implementing the EU's groundbreaking chemicals legislation for the benefit of human health and the environment as well as for innovation and competitiveness. | |
Explanation | Use of the information, documents and data from the ECHA website is subject to the terms and conditions of this Legal Notice, and subject to other binding limitations provided for under applicable law, the information, documents and data made available on the ECHA website may be reproduced, distributed and/or used, totally or in part, for non-commercial purposes provided that ECHA is acknowledged as the source: "Source: European Chemicals Agency, http://echa.europa.eu/". Such acknowledgement must be included in each copy of the material. ECHA permits and encourages organisations and individuals to create links to the ECHA website under the following cumulative conditions: Links can only be made to webpages that provide a link to the Legal Notice page. | |
Retrosynthesis Analysis
AI-Powered Synthesis Planning: Our tool employs the Template_relevance Pistachio, Template_relevance Bkms_metabolic, Template_relevance Pistachio_ringbreaker, Template_relevance Reaxys, Template_relevance Reaxys_biocatalysis model, leveraging a vast database of chemical reactions to predict feasible synthetic routes.
One-Step Synthesis Focus: Specifically designed for one-step synthesis, it provides concise and direct routes for your target compounds, streamlining the synthesis process.
Accurate Predictions: Utilizing the extensive PISTACHIO, BKMS_METABOLIC, PISTACHIO_RINGBREAKER, REAXYS, REAXYS_BIOCATALYSIS database, our tool offers high-accuracy predictions, reflecting the latest in chemical research and data.
Strategy Settings
Precursor scoring | Relevance Heuristic |
---|---|
Min. plausibility | 0.01 |
Model | Template_relevance |
Template Set | Pistachio/Bkms_metabolic/Pistachio_ringbreaker/Reaxys/Reaxys_biocatalysis |
Top-N result to add to graph | 6 |
Feasible Synthetic Routes
A: The research abstract highlights that 4-Chloro-3-fluorobenzonitrile acts as a key intermediate in the synthesis of 3,4-Difluorobenzonitrile. [] This suggests that during the halogen-exchange reaction, where 3,4-dichlorobenzonitrile reacts with potassium fluoride, the chlorine atom at the 4th position is preferentially replaced by fluorine, leading to the formation of this compound before complete fluorination to 3,4-Difluorobenzonitrile occurs.
Disclaimer and Information on In-Vitro Research Products
Please be aware that all articles and product information presented on BenchChem are intended solely for informational purposes. The products available for purchase on BenchChem are specifically designed for in-vitro studies, which are conducted outside of living organisms. In-vitro studies, derived from the Latin term "in glass," involve experiments performed in controlled laboratory settings using cells or tissues. It is important to note that these products are not categorized as medicines or drugs, and they have not received approval from the FDA for the prevention, treatment, or cure of any medical condition, ailment, or disease. We must emphasize that any form of bodily introduction of these products into humans or animals is strictly prohibited by law. It is essential to adhere to these guidelines to ensure compliance with legal and ethical standards in research and experimentation.