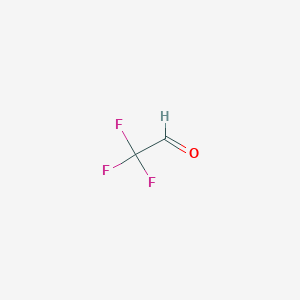
Trifluoroacetaldehyde
Overview
Description
Trifluoroacetaldehyde, also known as trifluoroethanal or fluoral, is a fluorinated derivative of acetaldehyde with the chemical formula CF₃CHO. It is a gas at room temperature and is known for its high reactivity and electrophilicity. This compound is primarily used to introduce trifluoromethyl groups into organic compounds, making it a valuable reagent in organic synthesis .
Synthetic Routes and Reaction Conditions:
Reduction of Trifluoroacetic Acid: this compound can be synthesized by reducing trifluoroacetic acid with lithium aluminium hydride in diethyl ether. The reaction proceeds as follows: [ \text{CF}_3\text{COOH} + \text{LiAlH}_4 \rightarrow \text{CF}_3\text{CHO} + \text{LiAlH}_3\text{O}_2 ]
Cathodic Reduction of Bromotrifluoromethane: Another method involves the cathodic reduction of bromotrifluoromethane in dimethylformamide with aluminium as the anode.
Vapour-Phase Oxidation of Trifluoroethanol: this compound can also be prepared by the vapour-phase oxidation of trifluoroethanol.
Industrial Production Methods:
Microwave-Assisted Preparation: Industrial production often employs microwave-assisted methods to enhance the efficiency and yield of this compound synthesis.
Types of Reactions:
Oxidation: this compound undergoes photolysis to produce fluoroform, hexafluoroethane, and carbon monoxide.
Reduction: It can be reduced to trifluoroethanol under specific conditions.
Substitution: this compound can participate in Mannich reactions to form trifluoromethyl-substituted heterocycles.
Common Reagents and Conditions:
Oxidation: Photolysis using ultraviolet light.
Reduction: Lithium aluminium hydride in diethyl ether.
Substitution: Formaldehydes and aromatic aldehydes in the presence of hydrazones.
Major Products:
- Fluoroform (CHF₃)
- Hexafluoroethane (C₂F₆)
- Carbon monoxide (CO)
- Trifluoromethyl-substituted heterocycles
Mechanism of Action
- Trifluoroacetaldehyde primarily interacts with various nucleophiles and electrophiles in chemical reactions. Its targets include functional groups such as carbonyl compounds, imines, and hydrazones .
Target of Action
Biochemical Pathways
Biochemical Analysis
Biochemical Properties
Trifluoroacetaldehyde is produced as a result of the reaction of HFO-1234ze with OH
Molecular Mechanism
It is known that this compound can undergo photodissociation to produce fluoroform (CHF 3 or HFC-23), a potent greenhouse gas
Temporal Effects in Laboratory Settings
In laboratory settings, this compound is studied for its atmospheric fate
Scientific Research Applications
Trifluoroacetaldehyde has a wide range of applications in scientific research:
- Chemistry: It is used as a reagent to introduce trifluoromethyl groups into organic molecules, which can significantly alter the chemical properties of the resulting compounds .
- Biology: this compound derivatives are used in the synthesis of biologically active molecules, including pharmaceuticals and agrochemicals .
- Medicine: It is employed in the development of new drugs, particularly those requiring the introduction of trifluoromethyl groups for enhanced bioactivity and metabolic stability .
- Industry: this compound is used in the production of refrigerants and blowing agents. It is also a key intermediate in the synthesis of various fluorinated compounds .
Comparison with Similar Compounds
- Chloral (CCl₃CHO)
- Bromal (CBr₃CHO)
- Iodal (CI₃CHO)
Comparison:
- Electrophilicity: Trifluoroacetaldehyde is highly electrophilic, similar to chloral, bromal, and iodal. the presence of fluorine atoms makes it more reactive and stable under certain conditions .
- Reactivity: this compound is more reactive than its chlorinated, brominated, and iodinated counterparts due to the strong electron-withdrawing effect of the fluorine atoms .
- Applications: While all these compounds are used to introduce halogenated groups into organic molecules, this compound is preferred for applications requiring high reactivity and stability .
Properties
IUPAC Name |
2,2,2-trifluoroacetaldehyde | |
---|---|---|
Source | PubChem | |
URL | https://pubchem.ncbi.nlm.nih.gov | |
Description | Data deposited in or computed by PubChem | |
InChI |
InChI=1S/C2HF3O/c3-2(4,5)1-6/h1H | |
Source | PubChem | |
URL | https://pubchem.ncbi.nlm.nih.gov | |
Description | Data deposited in or computed by PubChem | |
InChI Key |
JVTSHOJDBRTPHD-UHFFFAOYSA-N | |
Source | PubChem | |
URL | https://pubchem.ncbi.nlm.nih.gov | |
Description | Data deposited in or computed by PubChem | |
Canonical SMILES |
C(=O)C(F)(F)F | |
Source | PubChem | |
URL | https://pubchem.ncbi.nlm.nih.gov | |
Description | Data deposited in or computed by PubChem | |
Molecular Formula |
C2HF3O | |
Source | PubChem | |
URL | https://pubchem.ncbi.nlm.nih.gov | |
Description | Data deposited in or computed by PubChem | |
DSSTOX Substance ID |
DTXSID3058791 | |
Record name | Acetaldehyde, trifluoro- | |
Source | EPA DSSTox | |
URL | https://comptox.epa.gov/dashboard/DTXSID3058791 | |
Description | DSSTox provides a high quality public chemistry resource for supporting improved predictive toxicology. | |
Molecular Weight |
98.02 g/mol | |
Source | PubChem | |
URL | https://pubchem.ncbi.nlm.nih.gov | |
Description | Data deposited in or computed by PubChem | |
CAS No. |
75-90-1 | |
Record name | Trifluoroacetaldehyde | |
Source | CAS Common Chemistry | |
URL | https://commonchemistry.cas.org/detail?cas_rn=75-90-1 | |
Description | CAS Common Chemistry is an open community resource for accessing chemical information. Nearly 500,000 chemical substances from CAS REGISTRY cover areas of community interest, including common and frequently regulated chemicals, and those relevant to high school and undergraduate chemistry classes. This chemical information, curated by our expert scientists, is provided in alignment with our mission as a division of the American Chemical Society. | |
Explanation | The data from CAS Common Chemistry is provided under a CC-BY-NC 4.0 license, unless otherwise stated. | |
Record name | Trifluoroacetaldehyde | |
Source | ChemIDplus | |
URL | https://pubchem.ncbi.nlm.nih.gov/substance/?source=chemidplus&sourceid=0000075901 | |
Description | ChemIDplus is a free, web search system that provides access to the structure and nomenclature authority files used for the identification of chemical substances cited in National Library of Medicine (NLM) databases, including the TOXNET system. | |
Record name | Trifluoroacetaldehyde | |
Source | DTP/NCI | |
URL | https://dtp.cancer.gov/dtpstandard/servlet/dwindex?searchtype=NSC&outputformat=html&searchlist=9446 | |
Description | The NCI Development Therapeutics Program (DTP) provides services and resources to the academic and private-sector research communities worldwide to facilitate the discovery and development of new cancer therapeutic agents. | |
Explanation | Unless otherwise indicated, all text within NCI products is free of copyright and may be reused without our permission. Credit the National Cancer Institute as the source. | |
Record name | Acetaldehyde, 2,2,2-trifluoro- | |
Source | EPA Chemicals under the TSCA | |
URL | https://www.epa.gov/chemicals-under-tsca | |
Description | EPA Chemicals under the Toxic Substances Control Act (TSCA) collection contains information on chemicals and their regulations under TSCA, including non-confidential content from the TSCA Chemical Substance Inventory and Chemical Data Reporting. | |
Record name | Acetaldehyde, trifluoro- | |
Source | EPA DSSTox | |
URL | https://comptox.epa.gov/dashboard/DTXSID3058791 | |
Description | DSSTox provides a high quality public chemistry resource for supporting improved predictive toxicology. | |
Record name | Trifluoroacetaldehyde | |
Source | European Chemicals Agency (ECHA) | |
URL | https://echa.europa.eu/substance-information/-/substanceinfo/100.000.832 | |
Description | The European Chemicals Agency (ECHA) is an agency of the European Union which is the driving force among regulatory authorities in implementing the EU's groundbreaking chemicals legislation for the benefit of human health and the environment as well as for innovation and competitiveness. | |
Explanation | Use of the information, documents and data from the ECHA website is subject to the terms and conditions of this Legal Notice, and subject to other binding limitations provided for under applicable law, the information, documents and data made available on the ECHA website may be reproduced, distributed and/or used, totally or in part, for non-commercial purposes provided that ECHA is acknowledged as the source: "Source: European Chemicals Agency, http://echa.europa.eu/". Such acknowledgement must be included in each copy of the material. ECHA permits and encourages organisations and individuals to create links to the ECHA website under the following cumulative conditions: Links can only be made to webpages that provide a link to the Legal Notice page. | |
Retrosynthesis Analysis
AI-Powered Synthesis Planning: Our tool employs the Template_relevance Pistachio, Template_relevance Bkms_metabolic, Template_relevance Pistachio_ringbreaker, Template_relevance Reaxys, Template_relevance Reaxys_biocatalysis model, leveraging a vast database of chemical reactions to predict feasible synthetic routes.
One-Step Synthesis Focus: Specifically designed for one-step synthesis, it provides concise and direct routes for your target compounds, streamlining the synthesis process.
Accurate Predictions: Utilizing the extensive PISTACHIO, BKMS_METABOLIC, PISTACHIO_RINGBREAKER, REAXYS, REAXYS_BIOCATALYSIS database, our tool offers high-accuracy predictions, reflecting the latest in chemical research and data.
Strategy Settings
Precursor scoring | Relevance Heuristic |
---|---|
Min. plausibility | 0.01 |
Model | Template_relevance |
Template Set | Pistachio/Bkms_metabolic/Pistachio_ringbreaker/Reaxys/Reaxys_biocatalysis |
Top-N result to add to graph | 6 |
Feasible Synthetic Routes
Disclaimer and Information on In-Vitro Research Products
Please be aware that all articles and product information presented on BenchChem are intended solely for informational purposes. The products available for purchase on BenchChem are specifically designed for in-vitro studies, which are conducted outside of living organisms. In-vitro studies, derived from the Latin term "in glass," involve experiments performed in controlled laboratory settings using cells or tissues. It is important to note that these products are not categorized as medicines or drugs, and they have not received approval from the FDA for the prevention, treatment, or cure of any medical condition, ailment, or disease. We must emphasize that any form of bodily introduction of these products into humans or animals is strictly prohibited by law. It is essential to adhere to these guidelines to ensure compliance with legal and ethical standards in research and experimentation.