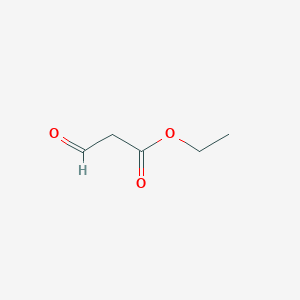
Ethyl 3-oxopropanoate
Overview
Description
Mechanism of Action
Ethyl 3-oxopropanoate, also known as Propanoic acid, 3-oxo-, ethyl ester or 3-Oxo-propionic acid ethyl ester, is a compound with the molecular formula C5H8O3 . This article will discuss its mechanism of action, biochemical pathways, pharmacokinetics, results of action, and the influence of environmental factors on its action.
Preparation Methods
Synthetic Routes and Reaction Conditions
3-Oxo-propionic acid ethyl ester can be synthesized through several methods. One common method involves the esterification of 3-oxopropanoic acid with ethanol in the presence of a strong acid catalyst such as sulfuric acid. The reaction is typically carried out under reflux conditions to ensure complete conversion .
Industrial Production Methods
In industrial settings, the production of 3-oxo-propionic acid ethyl ester often involves the use of continuous flow reactors to optimize yield and efficiency. The process may include steps such as distillation and purification to obtain the desired product with high purity .
Chemical Reactions Analysis
Types of Reactions
3-Oxo-propionic acid ethyl ester undergoes various chemical reactions, including:
Oxidation: It can be oxidized to form corresponding carboxylic acids.
Reduction: Reduction reactions can convert it into alcohols.
Substitution: It can participate in nucleophilic substitution reactions to form different esters or amides.
Common Reagents and Conditions
Oxidation: Common oxidizing agents include potassium permanganate and chromium trioxide.
Reduction: Reducing agents such as lithium aluminum hydride or sodium borohydride are often used.
Substitution: Nucleophiles like amines or alcohols can be used under acidic or basic conditions.
Major Products Formed
Oxidation: Produces carboxylic acids.
Reduction: Yields alcohols.
Substitution: Forms various esters or amides depending on the nucleophile used.
Scientific Research Applications
3-Oxo-propionic acid ethyl ester has a wide range of applications in scientific research:
Biology: It serves as a building block in the synthesis of biologically active molecules.
Medicine: It is used in the development of drugs and therapeutic agents.
Industry: It is employed in the production of flavors, fragrances, and other fine chemicals.
Comparison with Similar Compounds
Similar Compounds
Ethyl 3-oxobutyrate: Similar in structure but with an additional carbon atom.
Biological Activity
Ethyl 3-oxopropanoate, also known as ethyl acetoacetate, is a versatile compound in organic synthesis and medicinal chemistry. This article provides a detailed overview of its biological activity, including its mechanisms of action, therapeutic potentials, and relevant case studies.
Chemical Structure and Properties
This compound has the molecular formula C5H8O3. It features a ketone group adjacent to an ester, which contributes to its reactivity and biological properties. The compound is typically a colorless liquid with a fruity odor and is soluble in organic solvents.
Mechanisms of Biological Activity
This compound exhibits several biological activities, which can be attributed to its ability to interact with various molecular targets:
- Antimicrobial Activity : Studies have shown that this compound possesses significant antimicrobial properties against various bacterial strains. Its mechanism involves disrupting bacterial cell membranes and inhibiting essential metabolic pathways .
- Anticancer Properties : Research indicates that this compound can inhibit the proliferation of cancer cells. It appears to induce apoptosis in cancer cell lines by activating caspase pathways and inhibiting key enzymes involved in cell survival.
- Enzyme Inhibition : The compound has been reported to inhibit enzymes such as acetylcholinesterase, which plays a crucial role in neurotransmission. This property suggests potential applications in treating neurodegenerative diseases .
Case Study 1: Anticancer Activity
A study investigated the effects of this compound on human gastric cancer cell lines. The results demonstrated a dose-dependent reduction in cell viability, with IC50 values indicating significant cytotoxicity. The mechanism was linked to the induction of oxidative stress and subsequent apoptosis.
Concentration (µM) | Cell Viability (%) |
---|---|
0 | 100 |
10 | 85 |
50 | 60 |
100 | 30 |
Case Study 2: Antimicrobial Efficacy
Another study assessed the antimicrobial activity of this compound against Gram-positive and Gram-negative bacteria. The compound demonstrated minimum inhibitory concentrations (MICs) ranging from 125 µg/mL to 500 µg/mL, indicating its potential as an antimicrobial agent .
Bacterial Strain | MIC (µg/mL) |
---|---|
Staphylococcus aureus | 250 |
Escherichia coli | 500 |
Pseudomonas aeruginosa | 125 |
Research Findings
Recent research has focused on the synthesis of derivatives of this compound to enhance its biological activity. Modifications to the ester or ketone groups can lead to compounds with improved potency against specific targets.
Derivative Synthesis
Several derivatives have been synthesized and tested for enhanced biological activity:
- Ethyl 3-(4-chlorophenyl)amino-3-oxopropanoate : This derivative exhibited increased anticancer activity compared to the parent compound, suggesting that substituents can significantly influence biological outcomes.
Properties
IUPAC Name |
ethyl 3-oxopropanoate | |
---|---|---|
Source | PubChem | |
URL | https://pubchem.ncbi.nlm.nih.gov | |
Description | Data deposited in or computed by PubChem | |
InChI |
InChI=1S/C5H8O3/c1-2-8-5(7)3-4-6/h4H,2-3H2,1H3 | |
Source | PubChem | |
URL | https://pubchem.ncbi.nlm.nih.gov | |
Description | Data deposited in or computed by PubChem | |
InChI Key |
SYFFHRPDTQNMQB-UHFFFAOYSA-N | |
Source | PubChem | |
URL | https://pubchem.ncbi.nlm.nih.gov | |
Description | Data deposited in or computed by PubChem | |
Canonical SMILES |
CCOC(=O)CC=O | |
Source | PubChem | |
URL | https://pubchem.ncbi.nlm.nih.gov | |
Description | Data deposited in or computed by PubChem | |
Molecular Formula |
C5H8O3 | |
Source | PubChem | |
URL | https://pubchem.ncbi.nlm.nih.gov | |
Description | Data deposited in or computed by PubChem | |
DSSTOX Substance ID |
DTXSID60452420 | |
Record name | 3-Oxo-propionic acid ethyl ester | |
Source | EPA DSSTox | |
URL | https://comptox.epa.gov/dashboard/DTXSID60452420 | |
Description | DSSTox provides a high quality public chemistry resource for supporting improved predictive toxicology. | |
Molecular Weight |
116.11 g/mol | |
Source | PubChem | |
URL | https://pubchem.ncbi.nlm.nih.gov | |
Description | Data deposited in or computed by PubChem | |
CAS No. |
34780-29-5 | |
Record name | 3-Oxo-propionic acid ethyl ester | |
Source | EPA DSSTox | |
URL | https://comptox.epa.gov/dashboard/DTXSID60452420 | |
Description | DSSTox provides a high quality public chemistry resource for supporting improved predictive toxicology. | |
Retrosynthesis Analysis
AI-Powered Synthesis Planning: Our tool employs the Template_relevance Pistachio, Template_relevance Bkms_metabolic, Template_relevance Pistachio_ringbreaker, Template_relevance Reaxys, Template_relevance Reaxys_biocatalysis model, leveraging a vast database of chemical reactions to predict feasible synthetic routes.
One-Step Synthesis Focus: Specifically designed for one-step synthesis, it provides concise and direct routes for your target compounds, streamlining the synthesis process.
Accurate Predictions: Utilizing the extensive PISTACHIO, BKMS_METABOLIC, PISTACHIO_RINGBREAKER, REAXYS, REAXYS_BIOCATALYSIS database, our tool offers high-accuracy predictions, reflecting the latest in chemical research and data.
Strategy Settings
Precursor scoring | Relevance Heuristic |
---|---|
Min. plausibility | 0.01 |
Model | Template_relevance |
Template Set | Pistachio/Bkms_metabolic/Pistachio_ringbreaker/Reaxys/Reaxys_biocatalysis |
Top-N result to add to graph | 6 |
Feasible Synthetic Routes
Disclaimer and Information on In-Vitro Research Products
Please be aware that all articles and product information presented on BenchChem are intended solely for informational purposes. The products available for purchase on BenchChem are specifically designed for in-vitro studies, which are conducted outside of living organisms. In-vitro studies, derived from the Latin term "in glass," involve experiments performed in controlled laboratory settings using cells or tissues. It is important to note that these products are not categorized as medicines or drugs, and they have not received approval from the FDA for the prevention, treatment, or cure of any medical condition, ailment, or disease. We must emphasize that any form of bodily introduction of these products into humans or animals is strictly prohibited by law. It is essential to adhere to these guidelines to ensure compliance with legal and ethical standards in research and experimentation.