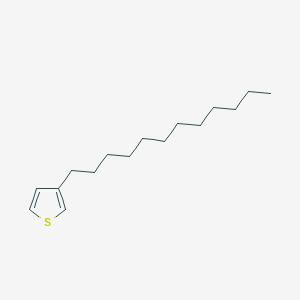
3-Dodecylthiophene
Overview
Description
3-Dodecylthiophene is an organic compound with the molecular formula C16H28S. It is a derivative of thiophene, where a dodecyl group is attached to the third carbon of the thiophene ring. This compound is known for its good electronic properties and is primarily used in the development of p-type semiconducting polymers. It is a conjugating monomer that can be used as an active layer on semiconductors .
Mechanism of Action
Target of Action
3-Dodecylthiophene (3-DT) is primarily used as a conjugating monomer in the development of p-type semiconducting polymers . It serves as an active layer on semiconductors .
Mode of Action
3-DT interacts with its targets by forming poly(this compound) (P3DT) through electrochemical polymerization . This process enhances the electronic properties of the resulting polymers, making them suitable for use in various organic electronic applications .
Biochemical Pathways
The primary biochemical pathway affected by 3-DT involves the formation of P3DT through electrochemical polymerization . The structures of the spin-coated P3DT thin films, along the out-of-plane and in-plane directions, are of immense importance for their performance as semiconducting materials .
Result of Action
The primary result of 3-DT’s action is the formation of P3DT, which exhibits good electronic properties and can be used in the development of p-type semiconducting polymers . These polymers can be utilized in a variety of organic electronic-based applications .
Action Environment
The action of 3-DT can be influenced by various environmental factors. For instance, the structures of the spin-coated P3DT thin films can be affected by solvent vapor annealing and thermal annealing . These processes can influence the performance of the resulting semiconducting materials .
Biochemical Analysis
Biochemical Properties
3-Dodecylthiophene is a small molecule that belongs to the class of thiophenes . It has been shown to have a number of interesting properties, including low energy fluorescence, UV absorption, and NMR spectra . It is also an important component in organic electronic devices such as polymer film, lasers, and molecular modeling .
Cellular Effects
The cellular effects of this compound are primarily observed in its role as a component of semiconducting polymers . For instance, multi-walled carbon nanotubes grafted with poly(this compound) (MWCNT-g-PDDT) have been used in perovskite solar cells to improve their morphological, optical, and photovoltaic properties .
Molecular Mechanism
The molecular mechanism of this compound primarily involves its role in the formation of semiconducting polymers . It is used in the formation of poly(this compound) (P3DT) through electrochemical polymerization
Temporal Effects in Laboratory Settings
Studies have shown that the orientation and crystallization of regioregular poly(this compound) (P3DDT) in different diameter nanopores can be influenced by solvent vapor annealing and thermal annealing .
Preparation Methods
Synthetic Routes and Reaction Conditions: 3-Dodecylthiophene can be synthesized from 3-bromothiophene and halogenated hydrocarbons in a single step. The reaction involves the use of magnesium turnings in anhydrous tetrahydrofuran (THF) under reflux conditions. The mixture is then cooled, and a nickel catalyst is added, followed by the slow addition of 3-bromothiophene .
Industrial Production Methods: In industrial settings, the preparation of this compound often involves similar methods but on a larger scale. The use of continuous flow reactors and automated systems ensures consistent quality and yield. The reaction conditions are optimized to maximize the production efficiency and minimize the formation of by-products .
Chemical Reactions Analysis
Types of Reactions: 3-Dodecylthiophene undergoes various chemical reactions, including:
Oxidation: It can be oxidized to form sulfoxides and sulfones.
Reduction: Reduction reactions can convert it into thiol derivatives.
Substitution: Electrophilic substitution reactions can introduce various functional groups onto the thiophene ring.
Common Reagents and Conditions:
Oxidation: Common oxidizing agents include hydrogen peroxide and m-chloroperbenzoic acid.
Reduction: Reducing agents such as lithium aluminum hydride are used.
Substitution: Reagents like bromine and iodine are used for halogenation reactions.
Major Products Formed:
Oxidation: Sulfoxides and sulfones.
Reduction: Thiol derivatives.
Substitution: Halogenated thiophenes.
Scientific Research Applications
3-Dodecylthiophene has a wide range of applications in scientific research:
Chemistry: It is used as a precursor for conducting polymers, particularly poly(this compound), which is used in organic electronic devices.
Biology: Its derivatives are studied for their potential biological activities.
Medicine: Research is ongoing to explore its use in drug delivery systems.
Industry: It is used in the production of semiconducting materials for organic field-effect transistors and photovoltaic cells
Comparison with Similar Compounds
- 3-Butylthiophene
- 3-Hexylthiophene
- 3-Octylthiophene
- 3-Decylthiophene
Comparison: 3-Dodecylthiophene is unique due to its longer alkyl chain, which provides better solubility and processability compared to its shorter-chain analogs. This makes it particularly useful in applications requiring high-performance semiconducting materials. The longer alkyl chain also enhances the thermal stability of the resulting polymers .
Properties
IUPAC Name |
3-dodecylthiophene | |
---|---|---|
Source | PubChem | |
URL | https://pubchem.ncbi.nlm.nih.gov | |
Description | Data deposited in or computed by PubChem | |
InChI |
InChI=1S/C16H28S/c1-2-3-4-5-6-7-8-9-10-11-12-16-13-14-17-15-16/h13-15H,2-12H2,1H3 | |
Source | PubChem | |
URL | https://pubchem.ncbi.nlm.nih.gov | |
Description | Data deposited in or computed by PubChem | |
InChI Key |
RFKWIEFTBMACPZ-UHFFFAOYSA-N | |
Source | PubChem | |
URL | https://pubchem.ncbi.nlm.nih.gov | |
Description | Data deposited in or computed by PubChem | |
Canonical SMILES |
CCCCCCCCCCCCC1=CSC=C1 | |
Source | PubChem | |
URL | https://pubchem.ncbi.nlm.nih.gov | |
Description | Data deposited in or computed by PubChem | |
Molecular Formula |
C16H28S | |
Source | PubChem | |
URL | https://pubchem.ncbi.nlm.nih.gov | |
Description | Data deposited in or computed by PubChem | |
Related CAS |
104934-53-4 | |
Record name | Poly(3-dodecylthiophene) | |
Source | CAS Common Chemistry | |
URL | https://commonchemistry.cas.org/detail?cas_rn=104934-53-4 | |
Description | CAS Common Chemistry is an open community resource for accessing chemical information. Nearly 500,000 chemical substances from CAS REGISTRY cover areas of community interest, including common and frequently regulated chemicals, and those relevant to high school and undergraduate chemistry classes. This chemical information, curated by our expert scientists, is provided in alignment with our mission as a division of the American Chemical Society. | |
Explanation | The data from CAS Common Chemistry is provided under a CC-BY-NC 4.0 license, unless otherwise stated. | |
DSSTOX Substance ID |
DTXSID40369961 | |
Record name | 3-Dodecylthiophene | |
Source | EPA DSSTox | |
URL | https://comptox.epa.gov/dashboard/DTXSID40369961 | |
Description | DSSTox provides a high quality public chemistry resource for supporting improved predictive toxicology. | |
Molecular Weight |
252.5 g/mol | |
Source | PubChem | |
URL | https://pubchem.ncbi.nlm.nih.gov | |
Description | Data deposited in or computed by PubChem | |
CAS No. |
104934-52-3 | |
Record name | 3-Dodecylthiophene | |
Source | EPA DSSTox | |
URL | https://comptox.epa.gov/dashboard/DTXSID40369961 | |
Description | DSSTox provides a high quality public chemistry resource for supporting improved predictive toxicology. | |
Record name | 3-(Dodec-1-yl)thiophene | |
Source | European Chemicals Agency (ECHA) | |
URL | https://echa.europa.eu/information-on-chemicals | |
Description | The European Chemicals Agency (ECHA) is an agency of the European Union which is the driving force among regulatory authorities in implementing the EU's groundbreaking chemicals legislation for the benefit of human health and the environment as well as for innovation and competitiveness. | |
Explanation | Use of the information, documents and data from the ECHA website is subject to the terms and conditions of this Legal Notice, and subject to other binding limitations provided for under applicable law, the information, documents and data made available on the ECHA website may be reproduced, distributed and/or used, totally or in part, for non-commercial purposes provided that ECHA is acknowledged as the source: "Source: European Chemicals Agency, http://echa.europa.eu/". Such acknowledgement must be included in each copy of the material. ECHA permits and encourages organisations and individuals to create links to the ECHA website under the following cumulative conditions: Links can only be made to webpages that provide a link to the Legal Notice page. | |
Synthesis routes and methods I
Procedure details
Synthesis routes and methods II
Procedure details
Retrosynthesis Analysis
AI-Powered Synthesis Planning: Our tool employs the Template_relevance Pistachio, Template_relevance Bkms_metabolic, Template_relevance Pistachio_ringbreaker, Template_relevance Reaxys, Template_relevance Reaxys_biocatalysis model, leveraging a vast database of chemical reactions to predict feasible synthetic routes.
One-Step Synthesis Focus: Specifically designed for one-step synthesis, it provides concise and direct routes for your target compounds, streamlining the synthesis process.
Accurate Predictions: Utilizing the extensive PISTACHIO, BKMS_METABOLIC, PISTACHIO_RINGBREAKER, REAXYS, REAXYS_BIOCATALYSIS database, our tool offers high-accuracy predictions, reflecting the latest in chemical research and data.
Strategy Settings
Precursor scoring | Relevance Heuristic |
---|---|
Min. plausibility | 0.01 |
Model | Template_relevance |
Template Set | Pistachio/Bkms_metabolic/Pistachio_ringbreaker/Reaxys/Reaxys_biocatalysis |
Top-N result to add to graph | 6 |
Feasible Synthetic Routes
Q1: What is the molecular formula and weight of 3-Dodecylthiophene?
A1: this compound has the molecular formula C16H28S and a molecular weight of 252.44 g/mol.
Q2: Are there any characteristic spectroscopic features of this compound?
A: Yes, poly(this compound) (P3DDT), the polymerized form of this compound, displays characteristic vibrational bands in the Cβ-H out-of-plane bending region of its FTIR spectrum. Specifically, the band at 827 cm−1 is assigned to the form II modification, the 806 cm−1 band to an intermediate phase with twisted main chains and ordered side chains, and the component at 800 cm−1 to doped segments. []
Q3: How does the choice of solvent affect the structure of poly(this compound) (P3DDT) thin films?
A: The solvent used to cast P3DDT thin films significantly impacts their structure. Films cast from toluene tend to exhibit better uniformity and ordering compared to those cast from chloroform or chlorobenzene. [] The rate of solvent evaporation also plays a role, with slower evaporation leading to better ordering in multilayer films. [] Additionally, the addition of a poor solvent, such as anisole, to a well-dissolved solution of P3DDT can induce the formation of nanowhiskers. []
Q4: Can the conductivity of P3DDT be affected by thermal treatment?
A: Yes, thermal dedoping of iodine-doped P3DDT can significantly affect its conductivity. Heating above 200°C can lead to a partial or complete loss of conductivity, likely due to structural changes and oxidation. [] This dedoping process appears irreversible at higher temperatures. []
Q5: How does blending P3DDT with low-density polyethylene (LDPE) affect its properties?
A: Blending P3DDT with LDPE leads to separate crystallizations of each component. In blends with low P3DDT content, P3DDT acts as a nucleating agent, influencing the crystal structure of LDPE. [] Doping these blends increases intermolecular forces, resulting in more compact packing and uniform P3DDT dispersion. [] Notably, blending improves the thermal stability of both P3DDT and LDPE, with a 5 wt% P3DDT blend exhibiting particularly enhanced stability. []
Q6: What is the impact of incorporating silicon nanowires into P3DDT films for field-effect transistor applications?
A: Adding silicon nanowires to P3DDT solutions before film formation significantly enhances the field-effect mobility of the resulting films. The silicon nanowires, acting as fast conducting channels between P3DDT's crystalline regions, lead to a remarkable increase in charge carrier mobility, from 0.015 cm2/Vs for pristine P3DDT films to up to 0.68 cm2/Vs for the blend films. [] This improvement is attributed to the high conductivity of the silicon nanowires. []
Q7: How does using an ion gel as the dielectric layer, compared to SiO2, impact the performance of P3DDT-based field-effect transistors?
A: Replacing the traditional SiO2 dielectric layer with an ion gel in P3DDT-based field-effect transistors leads to a substantial enhancement in charge carrier mobility. The mobility increases from 0.68 cm2/Vs for SiO2-based devices to 6.2 cm2/Vs for ion gel-based devices. [] This improvement is attributed to the enhanced dielectric properties of the ion gel. []
Q8: How does P3DDT interact with single-walled carbon nanotubes (SWNTs)?
A: P3DDT can non-covalently functionalize SWNTs, improving their solubility in various organic solvents like THF, dichlorobenzene, chloroform, and toluene. [] This interaction leads to a red-shift in the UV-Vis absorption maximum of P3DDT due to the planarization of its backbone upon adsorption onto the SWNT surface. [] The resulting P3DDT-SWNT complexes exhibit good solution stability even at elevated temperatures. []
Q9: What is the role of regioregularity in P3DDT-based block copolymers?
A: Regioregularity (RR) significantly impacts the self-assembly and crystallization behavior of P3DDT-based block copolymers. Higher RR leads to smaller domain spacings in both lamellar and cylindrical morphologies due to stronger crystallization and liquid crystalline interactions. [, ] Additionally, the crystallization mode is influenced by RR, with lower RR P3DDT crystallizing within glassy domains and high RR P3DDT crystallizing above the glass transition temperature, impacting the final morphology. []
Q10: How does P3DDT behave in dilute solutions?
A: In dilute solutions, regioregular P3DDT displays metastable aggregation under various conditions, including temperatures as high as 65 °C. [] Depending on factors like temperature and solvent, these aggregates can exhibit different shapes, such as disk-like or elongated structures, reflecting the complex interplay of intermolecular forces. [] These findings highlight the importance of considering thermal history when working with P3DDT solutions.
Q11: Can P3DDT be used in photovoltaic devices?
A: Yes, P3DDT shows potential for photovoltaic applications. For instance, a heterostructure composed of a diamond thin film and C60-doped P3DDT exhibited a quantum efficiency of 1.8% in the UV region. [, ] The diamond film acts as a protective, anti-reflective, and light-trapping layer, enhancing the device's performance. [, ]
Q12: What is the impact of the alkyl side chain length in poly(3-alkylthiophene)s on their crystallization and melting behavior?
A: The length of the alkyl side chain significantly affects the crystallization and melting behavior of poly(3-alkylthiophene)s. For instance, P3BT, P3OT, and P3DDT exhibit different equilibrium melting temperatures (Tm0) of 321, 230, and 175 °C, respectively. [] This trend highlights the influence of side chain length on intermolecular interactions and packing within the crystalline lattice.
Disclaimer and Information on In-Vitro Research Products
Please be aware that all articles and product information presented on BenchChem are intended solely for informational purposes. The products available for purchase on BenchChem are specifically designed for in-vitro studies, which are conducted outside of living organisms. In-vitro studies, derived from the Latin term "in glass," involve experiments performed in controlled laboratory settings using cells or tissues. It is important to note that these products are not categorized as medicines or drugs, and they have not received approval from the FDA for the prevention, treatment, or cure of any medical condition, ailment, or disease. We must emphasize that any form of bodily introduction of these products into humans or animals is strictly prohibited by law. It is essential to adhere to these guidelines to ensure compliance with legal and ethical standards in research and experimentation.