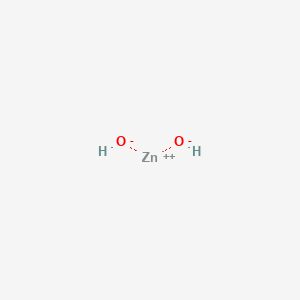
ZINC;dihydroxide
- Click on QUICK INQUIRY to receive a quote from our team of experts.
- With the quality product at a COMPETITIVE price, you can focus more on your research.
Overview
Description
Zinc dihydroxide, also known as zinc hydroxide, is an inorganic chemical compound with the chemical formula Zn(OH)₂. It is a white powder that is slightly soluble in water and insoluble in alcohol. Zinc dihydroxide occurs naturally as three rare minerals: wülfingite, ashoverite, and sweetite. This compound is amphoteric, meaning it can react with both acids and bases .
Preparation Methods
Synthetic Routes and Reaction Conditions: Zinc dihydroxide can be synthesized by dissolving zinc oxide in a concentrated aqueous solution of sodium hydroxide. The resulting solution is then strongly diluted, leading to the formation of zinc dihydroxide: [ \text{Zn}^{2+} + 2 \text{OH}^{-} \rightarrow \text{Zn(OH)}_2 ]
Industrial Production Methods: In industrial settings, zinc dihydroxide is often produced through the reaction of zinc salts with sodium hydroxide. The reaction is typically carried out at room temperature, and the product is then filtered, washed, and dried to obtain pure zinc dihydroxide .
Types of Reactions:
Oxidation and Reduction: Zinc dihydroxide can undergo oxidation to form zinc oxide (ZnO) when heated.
Substitution: It can react with acids to form zinc salts and water. For example, with hydrochloric acid, it forms zinc chloride and water: [ \text{Zn(OH)}_2 + 2 \text{HCl} \rightarrow \text{ZnCl}_2 + 2 \text{H}_2\text{O} ]
Common Reagents and Conditions:
Acids: Hydrochloric acid, sulfuric acid.
Bases: Sodium hydroxide, potassium hydroxide.
Ammonia: Zinc dihydroxide dissolves in excess aqueous ammonia to form a colorless, water-soluble ammine complex.
Major Products Formed:
Zinc oxide (ZnO): Formed upon heating.
Zinc salts: Formed through reactions with various acids.
Scientific Research Applications
Zinc dihydroxide has a wide range of applications in scientific research:
Chemistry: Used as a precursor for the synthesis of other zinc compounds.
Biology: Employed in the preparation of zinc-based catalysts and as a reagent in various biochemical assays.
Medicine: Utilized in the formulation of surgical dressings due to its absorbent properties.
Industry: Applied in the production of zinc salts and as an absorbent in various industrial processes .
Mechanism of Action
Zinc dihydroxide exerts its effects primarily through its amphoteric nature, allowing it to react with both acids and bases. In biological systems, zinc ions play crucial roles in enzyme function, protein synthesis, and cell division. Zinc dihydroxide can release zinc ions, which then participate in various biochemical pathways, including those involved in immune response and wound healing .
Comparison with Similar Compounds
Zinc oxide (ZnO): Similar in that it is also a zinc compound, but it is primarily used as a pigment and in sunscreens.
Cadmium hydroxide (Cd(OH)₂): Similar in structure but contains cadmium instead of zinc, making it more toxic and less commonly used.
Aluminium hydroxide (Al(OH)₃): Another amphoteric hydroxide, used mainly as an antacid and in water purification.
Uniqueness of Zinc Dihydroxide: Zinc dihydroxide’s amphoteric nature and its ability to form soluble complexes with ammonia distinguish it from other similar compounds. Its relatively low toxicity compared to cadmium compounds also makes it more suitable for various applications in medicine and industry .
Properties
IUPAC Name |
zinc;dihydroxide |
Source
|
---|---|---|
Source | PubChem | |
URL | https://pubchem.ncbi.nlm.nih.gov | |
Description | Data deposited in or computed by PubChem | |
InChI |
InChI=1S/2H2O.Zn/h2*1H2;/q;;+2/p-2 |
Source
|
Source | PubChem | |
URL | https://pubchem.ncbi.nlm.nih.gov | |
Description | Data deposited in or computed by PubChem | |
InChI Key |
UGZADUVQMDAIAO-UHFFFAOYSA-L |
Source
|
Source | PubChem | |
URL | https://pubchem.ncbi.nlm.nih.gov | |
Description | Data deposited in or computed by PubChem | |
Canonical SMILES |
[OH-].[OH-].[Zn+2] |
Source
|
Source | PubChem | |
URL | https://pubchem.ncbi.nlm.nih.gov | |
Description | Data deposited in or computed by PubChem | |
Molecular Formula |
H2O2Zn |
Source
|
Source | PubChem | |
URL | https://pubchem.ncbi.nlm.nih.gov | |
Description | Data deposited in or computed by PubChem | |
Molecular Weight |
99.4 g/mol |
Source
|
Source | PubChem | |
URL | https://pubchem.ncbi.nlm.nih.gov | |
Description | Data deposited in or computed by PubChem | |
CAS No. |
20427-58-1 |
Source
|
Record name | Zinc hydroxide | |
Source | CAS Common Chemistry | |
URL | https://commonchemistry.cas.org/detail?cas_rn=20427-58-1 | |
Description | CAS Common Chemistry is an open community resource for accessing chemical information. Nearly 500,000 chemical substances from CAS REGISTRY cover areas of community interest, including common and frequently regulated chemicals, and those relevant to high school and undergraduate chemistry classes. This chemical information, curated by our expert scientists, is provided in alignment with our mission as a division of the American Chemical Society. | |
Explanation | The data from CAS Common Chemistry is provided under a CC-BY-NC 4.0 license, unless otherwise stated. | |
Retrosynthesis Analysis
AI-Powered Synthesis Planning: Our tool employs the Template_relevance Pistachio, Template_relevance Bkms_metabolic, Template_relevance Pistachio_ringbreaker, Template_relevance Reaxys, Template_relevance Reaxys_biocatalysis model, leveraging a vast database of chemical reactions to predict feasible synthetic routes.
One-Step Synthesis Focus: Specifically designed for one-step synthesis, it provides concise and direct routes for your target compounds, streamlining the synthesis process.
Accurate Predictions: Utilizing the extensive PISTACHIO, BKMS_METABOLIC, PISTACHIO_RINGBREAKER, REAXYS, REAXYS_BIOCATALYSIS database, our tool offers high-accuracy predictions, reflecting the latest in chemical research and data.
Strategy Settings
Precursor scoring | Relevance Heuristic |
---|---|
Min. plausibility | 0.01 |
Model | Template_relevance |
Template Set | Pistachio/Bkms_metabolic/Pistachio_ringbreaker/Reaxys/Reaxys_biocatalysis |
Top-N result to add to graph | 6 |
Feasible Synthetic Routes
Q1: What is the role of zinc dihydroxide in the MOVPE growth of ZnO?
A1: Zinc dihydroxide is a key intermediate in the formation of ZnO during MOVPE. The process begins with diethylzinc (DEZn) reacting with oxygen atoms from nitrous oxide (N2O). This reaction forms diethoxy zinc (DEOZn; Zn(OC2H5)2). At high temperatures, DEOZn undergoes further reaction, eliminating ethylene (C2H4) and transforming into zinc dihydroxide []. This zinc dihydroxide then contributes to the formation of ZnO on the substrate.
Q2: Besides direct conversion to ZnO, are there other processes involving zinc dihydroxide during MOVPE?
A2: Yes, research indicates that oligomerization plays a significant role []. This means that alongside the main reaction pathway, reactants like zinc dihydroxide can also combine with themselves or other precursors to form dimers, trimers, and even larger oligomeric structures. These oligomers contribute to the overall deposition and growth of ZnO on the substrates.
Disclaimer and Information on In-Vitro Research Products
Please be aware that all articles and product information presented on BenchChem are intended solely for informational purposes. The products available for purchase on BenchChem are specifically designed for in-vitro studies, which are conducted outside of living organisms. In-vitro studies, derived from the Latin term "in glass," involve experiments performed in controlled laboratory settings using cells or tissues. It is important to note that these products are not categorized as medicines or drugs, and they have not received approval from the FDA for the prevention, treatment, or cure of any medical condition, ailment, or disease. We must emphasize that any form of bodily introduction of these products into humans or animals is strictly prohibited by law. It is essential to adhere to these guidelines to ensure compliance with legal and ethical standards in research and experimentation.