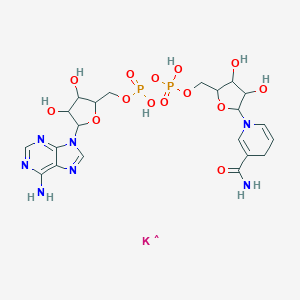
beta-Nicotinamide adenine dinucleotide, reduced dipotassium salt
- Click on QUICK INQUIRY to receive a quote from our team of experts.
- With the quality product at a COMPETITIVE price, you can focus more on your research.
Overview
Description
Beta-Nicotinamide adenine dinucleotide, reduced dipotassium salt is a useful research compound. Its molecular formula is C21H27K2N7O14P2 and its molecular weight is 741.6 g/mol. The purity is usually 95%.
BenchChem offers high-quality this compound suitable for many research applications. Different packaging options are available to accommodate customers' requirements. Please inquire for more information about this compound including the price, delivery time, and more detailed information at info@benchchem.com.
Mechanism of Action
Target of Action
Beta-Nicotinamide Adenine Dinucleotide, Reduced Dipotassium Salt (NADH), is a coenzyme that plays a crucial role in the oxidation-reduction reactions within cells . It primarily targets enzymes involved in these reactions, facilitating the transfer of electrons and hydrogen ions .
Mode of Action
NADH interacts with its targets by donating electrons, which are then used in various biochemical reactions . This electron transfer is fundamental to cellular energy production . Additionally, NADH acts as a donor of ADP-ribose units in ADP-ribosylation reactions .
Biochemical Pathways
NADH is involved in several biochemical pathways, including glycolysis, β-oxidation, and the citric acid cycle (also known as the Krebs cycle or TCA cycle) . These pathways are fundamental to cellular energy production . NADH also participates in cell signaling events, such as serving as a substrate for the poly (ADP-ribose) polymerases (PARPs) during the DNA damage response .
Pharmacokinetics
It’s known that nadh is an orally active compound .
Result of Action
The donation of electrons by NADH in redox reactions leads to the production of cellular energy . This energy is essential for the functioning of various biological processes. In addition, NADH’s role in ADP-ribosylation reactions and as a substrate for PARPs during the DNA damage response contributes to cellular signaling events .
Biochemical Analysis
Biochemical Properties
This compound is a key player in biochemical reactions. It functions as a regenerating electron donor in catabolic processes including glycolysis, β-oxidation, and the citric acid cycle (Krebs cycle, TCA cycle) . It interacts with various enzymes, proteins, and other biomolecules, facilitating the transfer of electrons in redox reactions that are fundamental to cellular energy production .
Cellular Effects
Beta-Nicotinamide adenine dinucleotide, reduced dipotassium salt, influences cell function in various ways. It participates in cell signaling events, for example, as a substrate for the poly (ADP-ribose) polymerases (PARPs) during the DNA damage response . It also plays a role in gene expression and cellular metabolism .
Molecular Mechanism
The molecular mechanism of this compound involves its role as an electron carrier. It accepts electrons from other molecules, becoming reduced to form NADH, which can then donate electrons in other reactions . This electron transfer is the main function of the compound. It also serves as a donor of ADP-ribose units in ADP-ribosylation reactions .
Metabolic Pathways
This compound, is involved in several metabolic pathways. It interacts with various enzymes and cofactors, and can affect metabolic flux or metabolite levels
Properties
CAS No. |
104809-32-7 |
---|---|
Molecular Formula |
C21H27K2N7O14P2 |
Molecular Weight |
741.6 g/mol |
IUPAC Name |
dipotassium;[[(2R,3S,4R,5R)-5-(6-aminopurin-9-yl)-3,4-dihydroxyoxolan-2-yl]methoxy-oxidophosphoryl] [(2R,3S,4R,5R)-5-(3-carbamoyl-4H-pyridin-1-yl)-3,4-dihydroxyoxolan-2-yl]methyl phosphate |
InChI |
InChI=1S/C21H29N7O14P2.2K/c22-17-12-19(25-7-24-17)28(8-26-12)21-16(32)14(30)11(41-21)6-39-44(36,37)42-43(34,35)38-5-10-13(29)15(31)20(40-10)27-3-1-2-9(4-27)18(23)33;;/h1,3-4,7-8,10-11,13-16,20-21,29-32H,2,5-6H2,(H2,23,33)(H,34,35)(H,36,37)(H2,22,24,25);;/q;2*+1/p-2/t10-,11-,13-,14-,15-,16-,20-,21-;;/m1../s1 |
InChI Key |
UMJWDCRRGAZCFU-WUEGHLCSSA-L |
SMILES |
C1C=CN(C=C1C(=O)N)C2C(C(C(O2)COP(=O)(O)OP(=O)(O)OCC3C(C(C(O3)N4C=NC5=C(N=CN=C54)N)O)O)O)O.[K] |
Isomeric SMILES |
C1C=CN(C=C1C(=O)N)[C@H]2[C@@H]([C@@H]([C@H](O2)COP(=O)([O-])OP(=O)([O-])OC[C@@H]3[C@H]([C@H]([C@@H](O3)N4C=NC5=C(N=CN=C54)N)O)O)O)O.[K+].[K+] |
Canonical SMILES |
C1C=CN(C=C1C(=O)N)C2C(C(C(O2)COP(=O)([O-])OP(=O)([O-])OCC3C(C(C(O3)N4C=NC5=C(N=CN=C54)N)O)O)O)O.[K+].[K+] |
Origin of Product |
United States |
Retrosynthesis Analysis
AI-Powered Synthesis Planning: Our tool employs the Template_relevance Pistachio, Template_relevance Bkms_metabolic, Template_relevance Pistachio_ringbreaker, Template_relevance Reaxys, Template_relevance Reaxys_biocatalysis model, leveraging a vast database of chemical reactions to predict feasible synthetic routes.
One-Step Synthesis Focus: Specifically designed for one-step synthesis, it provides concise and direct routes for your target compounds, streamlining the synthesis process.
Accurate Predictions: Utilizing the extensive PISTACHIO, BKMS_METABOLIC, PISTACHIO_RINGBREAKER, REAXYS, REAXYS_BIOCATALYSIS database, our tool offers high-accuracy predictions, reflecting the latest in chemical research and data.
Strategy Settings
Precursor scoring | Relevance Heuristic |
---|---|
Min. plausibility | 0.01 |
Model | Template_relevance |
Template Set | Pistachio/Bkms_metabolic/Pistachio_ringbreaker/Reaxys/Reaxys_biocatalysis |
Top-N result to add to graph | 6 |
Feasible Synthetic Routes
Disclaimer and Information on In-Vitro Research Products
Please be aware that all articles and product information presented on BenchChem are intended solely for informational purposes. The products available for purchase on BenchChem are specifically designed for in-vitro studies, which are conducted outside of living organisms. In-vitro studies, derived from the Latin term "in glass," involve experiments performed in controlled laboratory settings using cells or tissues. It is important to note that these products are not categorized as medicines or drugs, and they have not received approval from the FDA for the prevention, treatment, or cure of any medical condition, ailment, or disease. We must emphasize that any form of bodily introduction of these products into humans or animals is strictly prohibited by law. It is essential to adhere to these guidelines to ensure compliance with legal and ethical standards in research and experimentation.