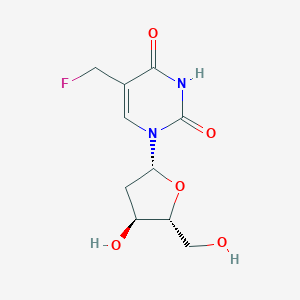
alpha-Fluorothymidine
- Click on QUICK INQUIRY to receive a quote from our team of experts.
- With the quality product at a COMPETITIVE price, you can focus more on your research.
Overview
Description
Alpha-Fluorothymidine, also known as Fluorothymidine F-18 (FLT), is a tumor-specific PET tracer and radiopharmaceutical . It is an isotopologue of alovudine . FLT is suitable for monitoring how tumors respond to cytostatic therapy . FLT accumulates in proliferating cells where it indicates the activity of the enzyme thymidine kinase .
Molecular Structure Analysis
Alpha-Fluorothymidine is a fluorinated tracer which has been proposed as an imaging biomarker of cell proliferation . It is a 18F-radiolabeled thymidine derivative and is taken up by proliferating cells .
Physical And Chemical Properties Analysis
The squaryl moiety in alpha-Fluorothymidine mimics the electrostatic properties of the phosphate group by forming resonance structures which result in two negatively charged carbonyls .
properties
IUPAC Name |
5-(fluoromethyl)-1-[(2R,4S,5R)-4-hydroxy-5-(hydroxymethyl)oxolan-2-yl]pyrimidine-2,4-dione |
Source
|
---|---|---|
Source | PubChem | |
URL | https://pubchem.ncbi.nlm.nih.gov | |
Description | Data deposited in or computed by PubChem | |
InChI |
InChI=1S/C10H13FN2O5/c11-2-5-3-13(10(17)12-9(5)16)8-1-6(15)7(4-14)18-8/h3,6-8,14-15H,1-2,4H2,(H,12,16,17)/t6-,7+,8+/m0/s1 |
Source
|
Source | PubChem | |
URL | https://pubchem.ncbi.nlm.nih.gov | |
Description | Data deposited in or computed by PubChem | |
InChI Key |
BQLWSXSBUAEINE-XLPZGREQSA-N |
Source
|
Source | PubChem | |
URL | https://pubchem.ncbi.nlm.nih.gov | |
Description | Data deposited in or computed by PubChem | |
Canonical SMILES |
C1C(C(OC1N2C=C(C(=O)NC2=O)CF)CO)O |
Source
|
Source | PubChem | |
URL | https://pubchem.ncbi.nlm.nih.gov | |
Description | Data deposited in or computed by PubChem | |
Isomeric SMILES |
C1[C@@H]([C@H](O[C@H]1N2C=C(C(=O)NC2=O)CF)CO)O |
Source
|
Source | PubChem | |
URL | https://pubchem.ncbi.nlm.nih.gov | |
Description | Data deposited in or computed by PubChem | |
Molecular Formula |
C10H13FN2O5 |
Source
|
Source | PubChem | |
URL | https://pubchem.ncbi.nlm.nih.gov | |
Description | Data deposited in or computed by PubChem | |
DSSTOX Substance ID |
DTXSID60906296 |
Source
|
Record name | 1-(2-Deoxypentofuranosyl)-5-(fluoromethyl)-4-hydroxypyrimidin-2(1H)-one | |
Source | EPA DSSTox | |
URL | https://comptox.epa.gov/dashboard/DTXSID60906296 | |
Description | DSSTox provides a high quality public chemistry resource for supporting improved predictive toxicology. | |
Molecular Weight |
260.22 g/mol |
Source
|
Source | PubChem | |
URL | https://pubchem.ncbi.nlm.nih.gov | |
Description | Data deposited in or computed by PubChem | |
Product Name |
alpha-Fluorothymidine | |
CAS RN |
101527-45-1 |
Source
|
Record name | alpha-Monofluorothymidine | |
Source | ChemIDplus | |
URL | https://pubchem.ncbi.nlm.nih.gov/substance/?source=chemidplus&sourceid=0101527451 | |
Description | ChemIDplus is a free, web search system that provides access to the structure and nomenclature authority files used for the identification of chemical substances cited in National Library of Medicine (NLM) databases, including the TOXNET system. | |
Record name | 1-(2-Deoxypentofuranosyl)-5-(fluoromethyl)-4-hydroxypyrimidin-2(1H)-one | |
Source | EPA DSSTox | |
URL | https://comptox.epa.gov/dashboard/DTXSID60906296 | |
Description | DSSTox provides a high quality public chemistry resource for supporting improved predictive toxicology. | |
Disclaimer and Information on In-Vitro Research Products
Please be aware that all articles and product information presented on BenchChem are intended solely for informational purposes. The products available for purchase on BenchChem are specifically designed for in-vitro studies, which are conducted outside of living organisms. In-vitro studies, derived from the Latin term "in glass," involve experiments performed in controlled laboratory settings using cells or tissues. It is important to note that these products are not categorized as medicines or drugs, and they have not received approval from the FDA for the prevention, treatment, or cure of any medical condition, ailment, or disease. We must emphasize that any form of bodily introduction of these products into humans or animals is strictly prohibited by law. It is essential to adhere to these guidelines to ensure compliance with legal and ethical standards in research and experimentation.