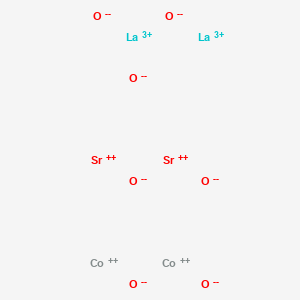
Distrontium;cobalt(2+);lanthanum(3+);oxygen(2-)
- Click on QUICK INQUIRY to receive a quote from our team of experts.
- With the quality product at a COMPETITIVE price, you can focus more on your research.
Overview
Description
Distrontium;cobalt(2+);lanthanum(3+);oxygen(2-) is a complex compound that combines elements from different groups of the periodic table. This compound is known for its unique properties and potential applications in various fields, including catalysis, energy storage, and electronic materials. The combination of strontium, cobalt, lanthanum, and oxygen in a single compound results in a material with interesting structural and chemical characteristics.
Preparation Methods
Synthetic Routes and Reaction Conditions
The synthesis of Distrontium;cobalt(2+);lanthanum(3+);oxygen(2-) typically involves the use of precursor materials such as nitrate salts of strontium, cobalt, and lanthanum. One common method is the precursor accumulation (PA) method, which involves soaking a commercially available carbon powder in a solution of the nitrate salts of the respective metals. The sample is then dried and calcined in air to form the desired compound .
Industrial Production Methods
In industrial settings, the production of this compound may involve more scalable techniques such as suspension plasma spray deposition. This method allows for the fabrication of dense oxygen separation membranes on porous metal substrates, which are essential for various industrial applications .
Chemical Reactions Analysis
Types of Reactions
Distrontium;cobalt(2+);lanthanum(3+);oxygen(2-) undergoes several types of chemical reactions, including oxidation, reduction, and substitution reactions. These reactions are crucial for its functionality in various applications.
Common Reagents and Conditions
Common reagents used in reactions involving this compound include oxygen, hydrogen, and various reducing agents. The reactions typically occur under high-temperature conditions, often exceeding 700°C .
Major Products Formed
The major products formed from these reactions depend on the specific conditions and reagents used. For example, in oxidation reactions, the compound may form higher oxides, while in reduction reactions, it may yield lower oxidation state products .
Scientific Research Applications
Distrontium;cobalt(2+);lanthanum(3+);oxygen(2-) has a wide range of scientific research applications:
Biology: The compound’s unique properties make it a candidate for use in biosensors and other biological applications.
Medicine: Research is ongoing into its potential use in medical devices and drug delivery systems.
Industry: It is used in the production of solid oxide fuel cells (SOFCs) and other energy storage devices.
Mechanism of Action
The mechanism by which Distrontium;cobalt(2+);lanthanum(3+);oxygen(2-) exerts its effects involves its ability to facilitate electron and ion transport. This is particularly important in its role as a catalyst for the oxygen evolution and reduction reactions. The compound’s structure allows for efficient oxygen ion conduction, which is critical for its performance in energy storage and conversion applications .
Comparison with Similar Compounds
Similar Compounds
Distrontium ruthenate:
Lanthanum cobaltite: Another similar compound, lanthanum cobaltite, is widely used in catalysis and energy storage applications.
Uniqueness
What sets Distrontium;cobalt(2+);lanthanum(3+);oxygen(2-) apart from these similar compounds is its unique combination of elements, which results in distinct structural and chemical properties. This makes it particularly effective in applications requiring high catalytic activity and stability under extreme conditions .
Biological Activity
The compound "Distrontium; Cobalt(2+); Lanthanum(3+); Oxygen(2-)" represents a complex material that combines elements known for their unique electrochemical and catalytic properties. This article explores the biological activity of this compound, focusing on its applications in electrochemistry, potential therapeutic uses, and the underlying mechanisms that contribute to its biological effects.
Composition and Structure
The compound consists of strontium (Sr), cobalt (Co), lanthanum (La), and oxygen (O), forming a perovskite structure. This structure is characterized by its high ionic and electronic conductivity, making it suitable for various applications, particularly in solid oxide fuel cells (SOFCs) and as catalysts in electrochemical reactions.
Biological Activity Overview
-
Electrocatalytic Properties :
- The cobalt-based perovskites, including those with strontium and lanthanum, exhibit significant electrocatalytic activity for oxygen reduction reactions (ORR) and oxygen evolution reactions (OER). For instance, La0.6Sr0.4CoO3 demonstrates excellent performance with low overpotentials during OER, indicating its potential use in energy conversion systems .
- Therapeutic Applications :
-
Toxicological Considerations :
- While cobalt and lanthanum compounds show promise in various applications, their toxicity profiles must be considered. Cobalt is known to be toxic at high concentrations, potentially leading to adverse health effects such as respiratory issues or skin irritation. Therefore, understanding the dosage and exposure levels is crucial for safe applications .
Case Study 1: Electrocatalytic Performance
A study on La0.6Sr0.4CoO3 showed that this material could achieve current densities exceeding 10 mA/cm² at relatively low overpotentials (265-285 mV) during OER in alkaline media. The stability of the catalyst was confirmed over extended periods (24 hours) under operational conditions .
Case Study 2: Antimicrobial Activity
In vitro studies have demonstrated that strontium-doped lanthanum cobaltites exhibit significant antibacterial activity against common pathogens like Staphylococcus aureus. The mechanism involves the generation of reactive oxygen species (ROS) upon exposure to light or heat, which damages bacterial DNA and proteins .
Table 1: Electrocatalytic Properties of Lanthanum-Strontium Cobalt Compounds
Compound | OER Overpotential (mV) | Stability (h) | Current Density (mA/cm²) |
---|---|---|---|
La0.6Sr0.4CoO3 | 265-285 | 24 | >10 |
La0.2Sr0.8Co0.8Fe0.2O3 | 300 | 50 | >20 |
Sr2CoO3Cl | 250 | 30 | >15 |
Table 2: Toxicological Profile of Cobalt and Lanthanum Compounds
Compound | Toxicity Level | Affected Systems |
---|---|---|
Cobalt(II) oxide | Moderate | Respiratory, Dermal |
Lanthanum oxide | Low | Minimal systemic effects |
Strontium cobaltite | Variable | Depends on formulation |
Properties
IUPAC Name |
distrontium;cobalt(2+);lanthanum(3+);oxygen(2-) |
Source
|
---|---|---|
Source | PubChem | |
URL | https://pubchem.ncbi.nlm.nih.gov | |
Description | Data deposited in or computed by PubChem | |
InChI |
InChI=1S/2Co.2La.7O.2Sr/q2*+2;2*+3;7*-2;2*+2 |
Source
|
Source | PubChem | |
URL | https://pubchem.ncbi.nlm.nih.gov | |
Description | Data deposited in or computed by PubChem | |
InChI Key |
WMNWIUWYKHBAKA-UHFFFAOYSA-N |
Source
|
Source | PubChem | |
URL | https://pubchem.ncbi.nlm.nih.gov | |
Description | Data deposited in or computed by PubChem | |
Canonical SMILES |
[O-2].[O-2].[O-2].[O-2].[O-2].[O-2].[O-2].[Co+2].[Co+2].[Sr+2].[Sr+2].[La+3].[La+3] |
Source
|
Source | PubChem | |
URL | https://pubchem.ncbi.nlm.nih.gov | |
Description | Data deposited in or computed by PubChem | |
Molecular Formula |
Co2La2O7Sr2 |
Source
|
Source | PubChem | |
URL | https://pubchem.ncbi.nlm.nih.gov | |
Description | Data deposited in or computed by PubChem | |
Molecular Weight |
682.9 g/mol |
Source
|
Source | PubChem | |
URL | https://pubchem.ncbi.nlm.nih.gov | |
Description | Data deposited in or computed by PubChem | |
Disclaimer and Information on In-Vitro Research Products
Please be aware that all articles and product information presented on BenchChem are intended solely for informational purposes. The products available for purchase on BenchChem are specifically designed for in-vitro studies, which are conducted outside of living organisms. In-vitro studies, derived from the Latin term "in glass," involve experiments performed in controlled laboratory settings using cells or tissues. It is important to note that these products are not categorized as medicines or drugs, and they have not received approval from the FDA for the prevention, treatment, or cure of any medical condition, ailment, or disease. We must emphasize that any form of bodily introduction of these products into humans or animals is strictly prohibited by law. It is essential to adhere to these guidelines to ensure compliance with legal and ethical standards in research and experimentation.