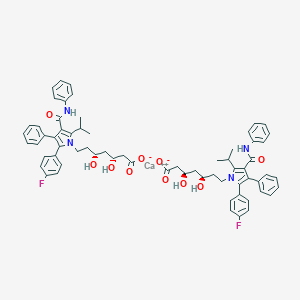
Atorvastatin calcium
Overview
Description
Atorvastatin calcium is a synthetic lipid-lowering agent belonging to the statin class of medications. It is primarily used to prevent cardiovascular diseases and treat abnormal lipid levels by inhibiting the enzyme 3-hydroxy-3-methylglutaryl-coenzyme A (HMG-CoA) reductase, which plays a crucial role in cholesterol production in the liver . This compound is widely known under the brand name Lipitor and has been one of the most commonly prescribed medications globally .
Mechanism of Action
Target of Action
Atorvastatin calcium, commonly known as Atorvastatin, is a lipid-lowering drug that belongs to the statin class of medications . Its primary target is the enzyme hydroxymethylglutaryl-coenzyme A (HMG-CoA) Reductase . This enzyme plays a crucial role in the endogenous production of cholesterol in the liver .
Mode of Action
Atorvastatin competitively inhibits HMG-CoA Reductase, which catalyzes the conversion of HMG-CoA to mevalonic acid . This conversion is a critical metabolic reaction involved in the production of several compounds involved in lipid metabolism and transport, including cholesterol, low-density lipoprotein (LDL), and very-low-density lipoprotein (VLDL) . By inhibiting this enzyme, Atorvastatin reduces the production of cholesterol, thereby lowering abnormal cholesterol and lipid levels .
Biochemical Pathways
The inhibition of HMG-CoA Reductase by Atorvastatin disrupts the mevalonate pathway, which is responsible for the de novo synthesis of cholesterol . This leads to a reduction in the levels of LDL-C and total cholesterol by 36-53% . In patients with dysbetalipoproteinemia, Atorvastatin reduces the levels of intermediate-density lipoprotein .
Pharmacokinetics
Atorvastatin is well absorbed orally but has low bioavailability . The liver metabolizes Atorvastatin through CYP3A4 enzymes and excretes it primarily in bile . The bioavailability of Atorvastatin can be enhanced by using certain techniques, such as solid dispersion, which can improve its solubility and dissolution rate .
Result of Action
The molecular and cellular effects of Atorvastatin’s action include a reduction in the risk of cardiovascular disease, including myocardial infarction and stroke . It also affects proliferation, migration, and survival of cancer cells . In addition, Atorvastatin has been shown to induce potent tumor-specific apoptosis .
Action Environment
Environmental factors can influence the action, efficacy, and stability of Atorvastatin. For instance, the solubility and bioavailability of Atorvastatin can be affected by the pH of the environment . Furthermore, certain environmental factors, such as exposure to UV light, can lead to the photodegradation of Atorvastatin .
Preparation Methods
Synthetic Routes and Reaction Conditions
The synthesis of atorvastatin calcium involves several key steps, including the construction of the pyrrole ring through methods such as Paal-Knorr condensation, [3+2] cycloaddition, or Hantzsch pyrrole synthesis . One prominent synthesis route involves the condensation of an elaborated 1,4-diketone with a fully protected side chain amine . The final stage involves the conversion of an advanced ketal ester intermediate to this compound, which includes ketal deprotection and ester hydrolysis followed by counter-ion exchange .
Industrial Production Methods
Industrial production of this compound has been optimized to achieve high yields and purity. Improvements include isolating the pure product of the ketal deprotection step as a crystalline solid and using ethyl acetate extraction to isolate pure this compound . Additionally, processes have been developed to produce this compound in amorphous form, which is stabilized and suitable for pharmaceutical use .
Chemical Reactions Analysis
Types of Reactions
Atorvastatin calcium undergoes various chemical reactions, including:
Hydrolysis: The compound can hydrolyze in the presence of aqueous solutions of NaOH, resulting in degradation products.
Common Reagents and Conditions
Oxidation: UV light exposure in the presence of water vapors and oxygen from the air.
Hydrolysis: Aqueous NaOH solutions.
Degradation: Acidic and basic conditions.
Major Products Formed
Photodegradation Products: Compounds with high C=O bond content.
Hydrolysis Products: Various degradation products identified through Raman scattering and FTIR spectroscopy.
Scientific Research Applications
Atorvastatin calcium has a wide range of scientific research applications:
Chemistry: Used in studies on drug stability, degradation kinetics, and photodegradation pathways
Biology: Investigated for its effects on cholesterol metabolism and cell membrane permeability.
Medicine: Widely used to lower lipid levels and reduce the risk of cardiovascular diseases, including myocardial infarction and stroke.
Comparison with Similar Compounds
Atorvastatin calcium is compared with other statins, such as lovastatin, pravastatin, rosuvastatin, fluvastatin, and simvastatin . While all statins share a common mechanism of action by inhibiting HMG-CoA reductase, this compound is unique in its high potency and ability to significantly reduce LDL cholesterol levels . Additionally, this compound has been shown to have a favorable safety profile and minimal side effects, making it a preferred choice for many patients .
Similar Compounds
- Lovastatin
- Pravastatin
- Rosuvastatin
- Fluvastatin
- Simvastatin
This compound stands out due to its high efficacy in reducing cholesterol levels and its extensive use in clinical practice .
Properties
CAS No. |
134523-03-8 |
---|---|
Molecular Formula |
C66H74CaF2N4O13 |
Molecular Weight |
1209.4 g/mol |
IUPAC Name |
calcium;(3R,5R)-7-[2-(4-fluorophenyl)-3-phenyl-4-(phenylcarbamoyl)-5-propan-2-ylpyrrol-1-yl]-3,5-dihydroxyheptanoate;trihydrate |
InChI |
InChI=1S/2C33H35FN2O5.Ca.3H2O/c2*1-21(2)31-30(33(41)35-25-11-7-4-8-12-25)29(22-9-5-3-6-10-22)32(23-13-15-24(34)16-14-23)36(31)18-17-26(37)19-27(38)20-28(39)40;;;;/h2*3-16,21,26-27,37-38H,17-20H2,1-2H3,(H,35,41)(H,39,40);;3*1H2/q;;+2;;;/p-2/t2*26-,27-;;;;/m11..../s1 |
InChI Key |
SHZPNDRIDUBNMH-NIJVSVLQSA-L |
SMILES |
CC(C)C1=C(C(=C(N1CCC(CC(CC(=O)[O-])O)O)C2=CC=C(C=C2)F)C3=CC=CC=C3)C(=O)NC4=CC=CC=C4.CC(C)C1=C(C(=C(N1CCC(CC(CC(=O)[O-])O)O)C2=CC=C(C=C2)F)C3=CC=CC=C3)C(=O)NC4=CC=CC=C4.[Ca+2] |
Isomeric SMILES |
CC(C)C1=C(C(=C(N1CC[C@H](C[C@H](CC(=O)[O-])O)O)C2=CC=C(C=C2)F)C3=CC=CC=C3)C(=O)NC4=CC=CC=C4.CC(C)C1=C(C(=C(N1CC[C@H](C[C@H](CC(=O)[O-])O)O)C2=CC=C(C=C2)F)C3=CC=CC=C3)C(=O)NC4=CC=CC=C4.O.O.O.[Ca+2] |
Canonical SMILES |
CC(C)C1=C(C(=C(N1CCC(CC(CC(=O)[O-])O)O)C2=CC=C(C=C2)F)C3=CC=CC=C3)C(=O)NC4=CC=CC=C4.CC(C)C1=C(C(=C(N1CCC(CC(CC(=O)[O-])O)O)C2=CC=C(C=C2)F)C3=CC=CC=C3)C(=O)NC4=CC=CC=C4.O.O.O.[Ca+2] |
Appearance |
Powder |
melting_point |
159.1 - 190.6 °C |
134523-03-8 | |
physical_description |
Solid |
Pictograms |
Irritant |
Related CAS |
110862-48-1 (Parent) |
solubility |
Practically insoluble |
Synonyms |
(3R,5R)-7-(2-(4-Fluorophenyl)-5-isopropyl-3-phenyl-4-(phenylcarbamoyl)-1H-pyrrol-1-yl)-3,5-dihydroxyheptanoic acid atorvastatin atorvastatin calcium atorvastatin calcium anhydrous atorvastatin calcium hydrate atorvastatin calcium trihydrate atorvastatin, calcium salt CI 981 CI-981 CI981 Lipitor liptonorm |
Origin of Product |
United States |
Retrosynthesis Analysis
AI-Powered Synthesis Planning: Our tool employs the Template_relevance Pistachio, Template_relevance Bkms_metabolic, Template_relevance Pistachio_ringbreaker, Template_relevance Reaxys, Template_relevance Reaxys_biocatalysis model, leveraging a vast database of chemical reactions to predict feasible synthetic routes.
One-Step Synthesis Focus: Specifically designed for one-step synthesis, it provides concise and direct routes for your target compounds, streamlining the synthesis process.
Accurate Predictions: Utilizing the extensive PISTACHIO, BKMS_METABOLIC, PISTACHIO_RINGBREAKER, REAXYS, REAXYS_BIOCATALYSIS database, our tool offers high-accuracy predictions, reflecting the latest in chemical research and data.
Strategy Settings
Precursor scoring | Relevance Heuristic |
---|---|
Min. plausibility | 0.01 |
Model | Template_relevance |
Template Set | Pistachio/Bkms_metabolic/Pistachio_ringbreaker/Reaxys/Reaxys_biocatalysis |
Top-N result to add to graph | 6 |
Feasible Synthetic Routes
Disclaimer and Information on In-Vitro Research Products
Please be aware that all articles and product information presented on BenchChem are intended solely for informational purposes. The products available for purchase on BenchChem are specifically designed for in-vitro studies, which are conducted outside of living organisms. In-vitro studies, derived from the Latin term "in glass," involve experiments performed in controlled laboratory settings using cells or tissues. It is important to note that these products are not categorized as medicines or drugs, and they have not received approval from the FDA for the prevention, treatment, or cure of any medical condition, ailment, or disease. We must emphasize that any form of bodily introduction of these products into humans or animals is strictly prohibited by law. It is essential to adhere to these guidelines to ensure compliance with legal and ethical standards in research and experimentation.