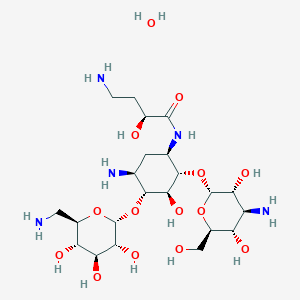
Amikacin hydrate
Overview
Description
Amikacin hydrate is a semi-synthetic aminoglycoside antibiotic derived from kanamycin A. It is primarily used to treat infections caused by resistant strains of Gram-negative bacteria and some Gram-positive bacteria . This compound is known for its effectiveness against bacteria such as Acinetobacter baumannii and Pseudomonas aeruginosa .
Scientific Research Applications
Amikacin hydrate has a wide range of scientific research applications:
Chemistry: Used in the study of aminoglycoside antibiotics and their chemical properties.
Biology: Employed in research on bacterial resistance and the development of new antibiotics.
Medicine: Utilized in the treatment of severe infections caused by multidrug-resistant bacteria.
Industry: Applied in the production of antibiotics and other pharmaceutical products.
Mechanism of Action
Amikacin hydrate exerts its effects by binding to the 30S ribosomal subunit of bacteria, interfering with mRNA binding and tRNA acceptor sites . This disruption leads to the production of non-functional or toxic proteins, ultimately inhibiting bacterial growth . The primary molecular targets are the bacterial ribosomes, and the pathways involved include protein synthesis inhibition .
Safety and Hazards
Future Directions
There is ongoing research into the use of Amikacin hydrate in combination with other substances, such as rutin hydrate, for increased antimicrobial potential . The introduction of natural compounds into treatment may increase the potential of Amikacin by improving its pharmacokinetic and pharmacodynamic properties, which could lead to a reduction in the doses, and thus reduce side effects associated with its use .
Biochemical Analysis
Biochemical Properties
Amikacin hydrate plays a crucial role in biochemical reactions by inhibiting protein synthesis in bacteria. It binds to the 30S and 50S ribosomal subunits, causing misreading of the mRNA sequence and inhibition of translocation . This interaction leads to the production of non-functional or toxic proteins, ultimately resulting in bacterial cell death . This compound interacts with various enzymes and proteins, including aminoglycoside-modifying enzymes, which can acetylate, phosphorylate, or adenylate the antibiotic, leading to resistance .
Cellular Effects
This compound exerts significant effects on various types of cells and cellular processes. It disrupts bacterial cell function by interfering with protein synthesis, which is essential for cell growth and replication . This disruption affects cell signaling pathways, gene expression, and cellular metabolism, leading to bacterial cell death . In mammalian cells, this compound can cause nephrotoxicity and ototoxicity, affecting kidney and auditory cells, respectively .
Molecular Mechanism
The primary mechanism of action of this compound involves binding to the bacterial 30S ribosomal subunit, which interferes with mRNA binding and tRNA acceptor sites . This binding disrupts normal protein synthesis, leading to the production of non-functional or toxic proteins . Additionally, this compound can inhibit the formation of the Z ring, a critical component of bacterial cell division . These molecular interactions result in the inhibition of bacterial growth and replication.
Temporal Effects in Laboratory Settings
In laboratory settings, the effects of this compound can change over time. The stability and degradation of this compound are influenced by factors such as temperature and pH . Long-term exposure to this compound can lead to the development of bacterial resistance due to the selection of resistant strains . In vitro and in vivo studies have shown that prolonged use of this compound can result in nephrotoxicity and ototoxicity, affecting kidney and auditory function .
Dosage Effects in Animal Models
The effects of this compound vary with different dosages in animal models. At therapeutic doses, this compound effectively treats bacterial infections without causing significant adverse effects . At high doses, this compound can cause nephrotoxicity and ototoxicity, leading to kidney damage and hearing loss . Threshold effects have been observed, where doses above a certain level result in toxic effects, while lower doses are well-tolerated .
Metabolic Pathways
This compound is primarily eliminated by the kidneys through glomerular filtration . Its structure has been modified to reduce enzymatic deactivation, thereby reducing bacterial resistance . This compound interacts with various metabolic pathways, including those involved in fatty acid metabolism, peptidoglycan synthesis, and lipopolysaccharide metabolism . These interactions can affect metabolic flux and metabolite levels, influencing bacterial survival and growth .
Transport and Distribution
This compound is transported and distributed within cells and tissues through various mechanisms. It is primarily distributed in the extracellular fluid and has limited penetration into cells . This compound interacts with transporters and binding proteins that facilitate its movement across cell membranes . The drug’s localization and accumulation are influenced by factors such as tissue perfusion and binding affinity .
Subcellular Localization
The subcellular localization of this compound is primarily in the cytoplasm, where it exerts its antibacterial effects by binding to ribosomal subunits . This compound does not have specific targeting signals or post-translational modifications that direct it to specific compartments or organelles . Its activity and function are largely dependent on its ability to reach and interact with bacterial ribosomes .
Preparation Methods
Amikacin hydrate is synthesized by acylation of the amino group of kanamycin A with L-(-)-γ-amino-α-hydroxybutyric acid . This process involves the reaction of kanamycin A with the acylating agent under controlled conditions to produce amikacin . Industrial production methods typically involve fermentation processes followed by chemical modification to achieve the desired compound .
Chemical Reactions Analysis
Amikacin hydrate undergoes several types of chemical reactions, including:
Common reagents used in these reactions include L-(-)-γ-amino-α-hydroxybutyric acid for acylation and various oxidizing agents for detection . The major products formed from these reactions are amikacin and its derivatives .
Comparison with Similar Compounds
Amikacin hydrate is unique among aminoglycosides due to its resistance to most aminoglycoside-modifying enzymes . Similar compounds include:
Kanamycin: The parent compound from which amikacin is derived.
Gentamicin: Another aminoglycoside antibiotic with a similar mechanism of action.
Neomycin: Used for similar bacterial infections but with different resistance profiles.
Amikacin’s uniqueness lies in its ability to treat infections caused by highly resistant bacteria, making it a valuable tool in combating antibiotic resistance .
properties
IUPAC Name |
(2S)-4-amino-N-[(1R,2S,3S,4R,5S)-5-amino-2-[(2S,3R,4S,5S,6R)-4-amino-3,5-dihydroxy-6-(hydroxymethyl)oxan-2-yl]oxy-4-[(2R,3R,4S,5S,6R)-6-(aminomethyl)-3,4,5-trihydroxyoxan-2-yl]oxy-3-hydroxycyclohexyl]-2-hydroxybutanamide;hydrate | |
---|---|---|
Source | PubChem | |
URL | https://pubchem.ncbi.nlm.nih.gov | |
Description | Data deposited in or computed by PubChem | |
InChI |
InChI=1S/C22H43N5O13.H2O/c23-2-1-8(29)20(36)27-7-3-6(25)18(39-22-16(34)15(33)13(31)9(4-24)37-22)17(35)19(7)40-21-14(32)11(26)12(30)10(5-28)38-21;/h6-19,21-22,28-35H,1-5,23-26H2,(H,27,36);1H2/t6-,7+,8-,9+,10+,11-,12+,13+,14+,15-,16+,17-,18+,19-,21+,22+;/m0./s1 | |
Source | PubChem | |
URL | https://pubchem.ncbi.nlm.nih.gov | |
Description | Data deposited in or computed by PubChem | |
InChI Key |
DTSOZYYWEZJFSS-XTHCGPPUSA-N | |
Source | PubChem | |
URL | https://pubchem.ncbi.nlm.nih.gov | |
Description | Data deposited in or computed by PubChem | |
Canonical SMILES |
C1C(C(C(C(C1NC(=O)C(CCN)O)OC2C(C(C(C(O2)CO)O)N)O)O)OC3C(C(C(C(O3)CN)O)O)O)N.O | |
Source | PubChem | |
URL | https://pubchem.ncbi.nlm.nih.gov | |
Description | Data deposited in or computed by PubChem | |
Isomeric SMILES |
C1[C@@H]([C@H]([C@@H]([C@H]([C@@H]1NC(=O)[C@H](CCN)O)O[C@@H]2[C@@H]([C@H]([C@@H]([C@H](O2)CO)O)N)O)O)O[C@@H]3[C@@H]([C@H]([C@@H]([C@H](O3)CN)O)O)O)N.O | |
Source | PubChem | |
URL | https://pubchem.ncbi.nlm.nih.gov | |
Description | Data deposited in or computed by PubChem | |
Molecular Formula |
C22H45N5O14 | |
Source | PubChem | |
URL | https://pubchem.ncbi.nlm.nih.gov | |
Description | Data deposited in or computed by PubChem | |
Molecular Weight |
603.6 g/mol | |
Source | PubChem | |
URL | https://pubchem.ncbi.nlm.nih.gov | |
Description | Data deposited in or computed by PubChem | |
CAS RN |
1257517-67-1 | |
Record name | D-Streptamine, O-3-amino-3-deoxy-α-D-glucopyranosyl-(1→6)-O-[6-amino-6-deoxy-α-D-glucopyranosyl-(1→4)]-N1-[(2S)-4-amino-2-hydroxy-1-oxobutyl]-2-deoxy-, hydrate (1:?) | |
Source | CAS Common Chemistry | |
URL | https://commonchemistry.cas.org/detail?cas_rn=1257517-67-1 | |
Description | CAS Common Chemistry is an open community resource for accessing chemical information. Nearly 500,000 chemical substances from CAS REGISTRY cover areas of community interest, including common and frequently regulated chemicals, and those relevant to high school and undergraduate chemistry classes. This chemical information, curated by our expert scientists, is provided in alignment with our mission as a division of the American Chemical Society. | |
Explanation | The data from CAS Common Chemistry is provided under a CC-BY-NC 4.0 license, unless otherwise stated. | |
Retrosynthesis Analysis
AI-Powered Synthesis Planning: Our tool employs the Template_relevance Pistachio, Template_relevance Bkms_metabolic, Template_relevance Pistachio_ringbreaker, Template_relevance Reaxys, Template_relevance Reaxys_biocatalysis model, leveraging a vast database of chemical reactions to predict feasible synthetic routes.
One-Step Synthesis Focus: Specifically designed for one-step synthesis, it provides concise and direct routes for your target compounds, streamlining the synthesis process.
Accurate Predictions: Utilizing the extensive PISTACHIO, BKMS_METABOLIC, PISTACHIO_RINGBREAKER, REAXYS, REAXYS_BIOCATALYSIS database, our tool offers high-accuracy predictions, reflecting the latest in chemical research and data.
Strategy Settings
Precursor scoring | Relevance Heuristic |
---|---|
Min. plausibility | 0.01 |
Model | Template_relevance |
Template Set | Pistachio/Bkms_metabolic/Pistachio_ringbreaker/Reaxys/Reaxys_biocatalysis |
Top-N result to add to graph | 6 |
Feasible Synthetic Routes
Q & A
Q1: What novel target has been explored for Amikacin Hydrate in the context of tuberculosis treatment? []
A1: Recent computational studies suggest that this compound could potentially inhibit Mycobacterium tuberculosis (Mtb) resuscitation from a dormant state by targeting resuscitation-promoting factor B (RpfB). [] This protein plays a crucial role in reactivating dormant Mtb, a major factor contributing to the spread of tuberculosis, especially latent tuberculosis infection. []
Q2: How does this compound compare to other potential RpfB inhibitors in silico? []
A2: Computational modeling indicates that this compound exhibits strong binding affinity to the active site of RpfB, with a binding free energy of -66.87 kcal/mol. [] This binding affinity surpasses that of the reference inhibitor, 4-benzoyl-2-nitrophenyl thiocyanate (NPT7). [] Molecular dynamics simulations further suggest that this compound forms a stable complex with RpfB, potentially hindering its function. []
Q3: Besides its antibacterial activity, has this compound been explored for other applications in disease modeling? []
A3: Yes, this compound has been employed in the development of novel hydrogel systems named "Amikagels" for creating three-dimensional tumor microenvironments (3DTMs) of breast cancer cells. [] These 3DTMs, generated by crosslinking this compound with poly(ethylene glycol) diglycidyl ether, provide a more physiologically relevant model for studying tumor behavior and drug resistance compared to traditional two-dimensional cell cultures. []
Q4: What are the implications of using this compound in generating 3DTMs for drug discovery? []
A4: Utilizing this compound-based hydrogels to create 3DTMs offers a valuable platform for studying drug resistance mechanisms in cancer. [] For instance, breast cancer 3DTMs grown on Amikagels exhibited increased resistance to chemotherapeutic drugs like mitoxantrone and doxorubicin compared to their 2D counterparts. [] This highlights the potential of such models in identifying novel therapeutic targets and developing more effective treatment strategies. []
Disclaimer and Information on In-Vitro Research Products
Please be aware that all articles and product information presented on BenchChem are intended solely for informational purposes. The products available for purchase on BenchChem are specifically designed for in-vitro studies, which are conducted outside of living organisms. In-vitro studies, derived from the Latin term "in glass," involve experiments performed in controlled laboratory settings using cells or tissues. It is important to note that these products are not categorized as medicines or drugs, and they have not received approval from the FDA for the prevention, treatment, or cure of any medical condition, ailment, or disease. We must emphasize that any form of bodily introduction of these products into humans or animals is strictly prohibited by law. It is essential to adhere to these guidelines to ensure compliance with legal and ethical standards in research and experimentation.