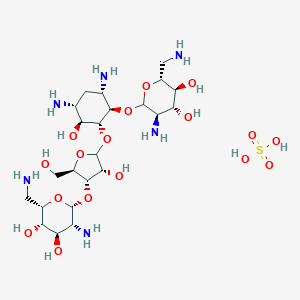
Framycetin sulfate
Overview
Description
Framycetin sulfate, also known as Neomycin B sulfate, is an aminoglycoside antibiotic . It is used in various formulations and combinations with other agents to treat hemorrhoids, eye and ear infections, and to reduce nasal carriage of staphylococcus . It is produced by Streptomyces fradiae and on hydrolysis, it yields neamine and neobiosamine B .
Molecular Structure Analysis
Framycetin sulfate is a small molecule with the chemical formula C23H46N6O13 . Its average molecular weight is 614.6437 .Physical And Chemical Properties Analysis
Framycetin sulfate is a solid substance that is soluble in water . Its molecular weight is 712.72 .Scientific Research Applications
Microbial Research
Neomycin Sulfate is used in microbial research to improve the potency of Streptomyces fradiae strains. A high-throughput screening method using streptomycin resistance prescreening and a 24-deep well plates/microplate reader rescreening strategy was developed. This approach identified a high-producing Neomycin mutant strain .
Bacterial Contamination Prevention
Neomycin Sulfate is used for the prevention of bacterial contamination of cell cultures. This antibiotic is highly active against gram-negative bacteria .
Molecular Biology Applications
Neomycin is often used as a selection agent in molecular biology applications for the nptII gene .
Phospholipase C Inhibition
Neomycin sulfate inhibits Phospholipase C via binding to inositol phospholipids. It also inhibits phosphatidylcholine-PLD activity and induces Ca2+ mobilization and PLA2 activation in human platelets .
Buccal Mucosal Wound Healing
Framycetin sulfate is used in the formulation of gels loaded with solid lipid nanoparticles for buccal mucosal wound healing .
Ocular In Situ Gel Formulation
Framycetin sulfate is used in the formulation of ocular in situ gels for the treatment of ocular eye infections. It is formulated as an ocular in situ gel forming eye drop using polymers such as carbopol 940 as a gelling agent in combination with suitable preservatives .
Treatment of Bacterial Eye Infections
Framycetin is used for the treatment of bacterial eye infections such as conjunctivitis. It works by binding to the bacterial 30S ribosomal subunit, causing misreading of t-RNA, leaving the bacterium unable to synthesize proteins vital to its growth .
Topical Gel for Wound Healing
Framycetin sulfate is used in the formulation of microsponge loaded topical gels for the treatment of wound healing .
Mechanism of Action
Target of Action
Neomycin sulfate, also known as Framycetin sulfate, is an aminoglycoside antibiotic derived from the metabolic products of Streptomyces fradiae . It primarily targets bacterial ribosomes , which are crucial for protein synthesis and survival of bacteria .
Mode of Action
Neomycin sulfate exerts its pharmacological effects by binding to bacterial ribosomes and inhibiting protein synthesis . This interaction disrupts protein synthesis, which is essential for bacterial growth and survival .
Biochemical Pathways
Neomycin sulfate affects the hexose monophosphate (HMP) pathway . It enhances the expression of neo genes involved in the neomycin B biosynthesis pathway . The addition of inorganic salts such as ammonium sulfate can promote neomycin B biosynthesis and cell growth .
Pharmacokinetics
It is also used in combination with other compounds in otic suspensions for treating bacterial infections in the external auditory canal .
Result of Action
The primary result of neomycin sulfate’s action is the suppression of bacterial growth and survival . By inhibiting protein synthesis, it disrupts the normal functioning of bacteria, leading to their death .
Action Environment
Environmental factors can influence the action of neomycin sulfate. For instance, the presence of inorganic salts like ammonium sulfate can enhance neomycin B biosynthesis . Additionally, the character of the microbial flora in the intestine may influence the effectiveness of neomycin sulfate .
Safety and Hazards
Framycetin sulfate is toxic and should be handled only by personnel trained and familiar with handling potent active pharmaceutical ingredients . It is a moderate to severe irritant to the skin and eyes . It is advised to avoid dust formation, breathing mist, gas or vapors, and contact with skin and eye . Use personal protective equipment, ensure adequate ventilation, remove all sources of ignition, and evacuate personnel to safe areas .
properties
IUPAC Name |
(2R,3S,4R,5R,6R)-5-amino-2-(aminomethyl)-6-[(1R,2R,3S,4R,6S)-4,6-diamino-2-[(2S,3R,4S,5R)-4-[(2R,3R,4R,5S,6S)-3-amino-6-(aminomethyl)-4,5-dihydroxyoxan-2-yl]oxy-3-hydroxy-5-(hydroxymethyl)oxolan-2-yl]oxy-3-hydroxycyclohexyl]oxyoxane-3,4-diol;sulfuric acid | |
---|---|---|
Source | PubChem | |
URL | https://pubchem.ncbi.nlm.nih.gov | |
Description | Data deposited in or computed by PubChem | |
InChI |
InChI=1S/C23H46N6O13.H2O4S/c24-2-7-13(32)15(34)10(28)21(37-7)40-18-6(27)1-5(26)12(31)20(18)42-23-17(36)19(9(4-30)39-23)41-22-11(29)16(35)14(33)8(3-25)38-22;1-5(2,3)4/h5-23,30-36H,1-4,24-29H2;(H2,1,2,3,4)/t5-,6+,7-,8+,9-,10-,11-,12+,13-,14-,15-,16-,17-,18-,19-,20-,21-,22-,23+;/m1./s1 | |
Source | PubChem | |
URL | https://pubchem.ncbi.nlm.nih.gov | |
Description | Data deposited in or computed by PubChem | |
InChI Key |
OIXVKQDWLFHVGR-WQDIDPJDSA-N | |
Source | PubChem | |
URL | https://pubchem.ncbi.nlm.nih.gov | |
Description | Data deposited in or computed by PubChem | |
Canonical SMILES |
C1C(C(C(C(C1N)OC2C(C(C(C(O2)CN)O)O)N)OC3C(C(C(O3)CO)OC4C(C(C(C(O4)CN)O)O)N)O)O)N.OS(=O)(=O)O | |
Source | PubChem | |
URL | https://pubchem.ncbi.nlm.nih.gov | |
Description | Data deposited in or computed by PubChem | |
Isomeric SMILES |
C1[C@H]([C@@H]([C@H]([C@@H]([C@H]1N)O[C@@H]2[C@@H]([C@H]([C@@H]([C@H](O2)CN)O)O)N)O[C@H]3[C@@H]([C@@H]([C@H](O3)CO)O[C@@H]4[C@@H]([C@H]([C@@H]([C@@H](O4)CN)O)O)N)O)O)N.OS(=O)(=O)O | |
Source | PubChem | |
URL | https://pubchem.ncbi.nlm.nih.gov | |
Description | Data deposited in or computed by PubChem | |
Molecular Formula |
C23H48N6O17S | |
Source | PubChem | |
URL | https://pubchem.ncbi.nlm.nih.gov | |
Description | Data deposited in or computed by PubChem | |
Related CAS |
25389-98-4 | |
Record name | D-Streptamine, O-2,6-diamino-2,6-dideoxy-β-L-idopyranosyl-(1→3)-O-β-D-ribofuranosyl-(1→5)-O-[2,6-diamino-2,6-dideoxy-α-D-glucopyranosyl-(1→4)]-2-deoxy-, sulfate (1:1) | |
Source | CAS Common Chemistry | |
URL | https://commonchemistry.cas.org/detail?cas_rn=25389-98-4 | |
Description | CAS Common Chemistry is an open community resource for accessing chemical information. Nearly 500,000 chemical substances from CAS REGISTRY cover areas of community interest, including common and frequently regulated chemicals, and those relevant to high school and undergraduate chemistry classes. This chemical information, curated by our expert scientists, is provided in alignment with our mission as a division of the American Chemical Society. | |
Explanation | The data from CAS Common Chemistry is provided under a CC-BY-NC 4.0 license, unless otherwise stated. | |
DSSTOX Substance ID |
DTXSID10948247 | |
Record name | Neomycin B sulfate | |
Source | EPA DSSTox | |
URL | https://comptox.epa.gov/dashboard/DTXSID10948247 | |
Description | DSSTox provides a high quality public chemistry resource for supporting improved predictive toxicology. | |
Molecular Weight |
712.7 g/mol | |
Source | PubChem | |
URL | https://pubchem.ncbi.nlm.nih.gov | |
Description | Data deposited in or computed by PubChem | |
Product Name |
Framycetin sulfate | |
CAS RN |
4146-30-9, 28002-70-2, 1405-10-3, 25389-98-4 | |
Record name | Neomycin B sulfate | |
Source | CAS Common Chemistry | |
URL | https://commonchemistry.cas.org/detail?cas_rn=4146-30-9 | |
Description | CAS Common Chemistry is an open community resource for accessing chemical information. Nearly 500,000 chemical substances from CAS REGISTRY cover areas of community interest, including common and frequently regulated chemicals, and those relevant to high school and undergraduate chemistry classes. This chemical information, curated by our expert scientists, is provided in alignment with our mission as a division of the American Chemical Society. | |
Explanation | The data from CAS Common Chemistry is provided under a CC-BY-NC 4.0 license, unless otherwise stated. | |
Record name | Framycetin sulfate | |
Source | CAS Common Chemistry | |
URL | https://commonchemistry.cas.org/detail?cas_rn=28002-70-2 | |
Description | CAS Common Chemistry is an open community resource for accessing chemical information. Nearly 500,000 chemical substances from CAS REGISTRY cover areas of community interest, including common and frequently regulated chemicals, and those relevant to high school and undergraduate chemistry classes. This chemical information, curated by our expert scientists, is provided in alignment with our mission as a division of the American Chemical Society. | |
Explanation | The data from CAS Common Chemistry is provided under a CC-BY-NC 4.0 license, unless otherwise stated. | |
Record name | Neomycin B, sulfate (salt) | |
Source | ChemIDplus | |
URL | https://pubchem.ncbi.nlm.nih.gov/substance/?source=chemidplus&sourceid=0028002702 | |
Description | ChemIDplus is a free, web search system that provides access to the structure and nomenclature authority files used for the identification of chemical substances cited in National Library of Medicine (NLM) databases, including the TOXNET system. | |
Record name | Neomycin, sulfate (salt) | |
Source | EPA Chemicals under the TSCA | |
URL | https://www.epa.gov/chemicals-under-tsca | |
Description | EPA Chemicals under the Toxic Substances Control Act (TSCA) collection contains information on chemicals and their regulations under TSCA, including non-confidential content from the TSCA Chemical Substance Inventory and Chemical Data Reporting. | |
Record name | Neomycin B sulfate | |
Source | EPA DSSTox | |
URL | https://comptox.epa.gov/dashboard/DTXSID10948247 | |
Description | DSSTox provides a high quality public chemistry resource for supporting improved predictive toxicology. | |
Record name | Framycetin sulphate | |
Source | European Chemicals Agency (ECHA) | |
URL | https://echa.europa.eu/substance-information/-/substanceinfo/100.021.791 | |
Description | The European Chemicals Agency (ECHA) is an agency of the European Union which is the driving force among regulatory authorities in implementing the EU's groundbreaking chemicals legislation for the benefit of human health and the environment as well as for innovation and competitiveness. | |
Explanation | Use of the information, documents and data from the ECHA website is subject to the terms and conditions of this Legal Notice, and subject to other binding limitations provided for under applicable law, the information, documents and data made available on the ECHA website may be reproduced, distributed and/or used, totally or in part, for non-commercial purposes provided that ECHA is acknowledged as the source: "Source: European Chemicals Agency, http://echa.europa.eu/". Such acknowledgement must be included in each copy of the material. ECHA permits and encourages organisations and individuals to create links to the ECHA website under the following cumulative conditions: Links can only be made to webpages that provide a link to the Legal Notice page. | |
Record name | Neomycin B sulphate | |
Source | European Chemicals Agency (ECHA) | |
URL | https://echa.europa.eu/substance-information/-/substanceinfo/100.044.322 | |
Description | The European Chemicals Agency (ECHA) is an agency of the European Union which is the driving force among regulatory authorities in implementing the EU's groundbreaking chemicals legislation for the benefit of human health and the environment as well as for innovation and competitiveness. | |
Explanation | Use of the information, documents and data from the ECHA website is subject to the terms and conditions of this Legal Notice, and subject to other binding limitations provided for under applicable law, the information, documents and data made available on the ECHA website may be reproduced, distributed and/or used, totally or in part, for non-commercial purposes provided that ECHA is acknowledged as the source: "Source: European Chemicals Agency, http://echa.europa.eu/". Such acknowledgement must be included in each copy of the material. ECHA permits and encourages organisations and individuals to create links to the ECHA website under the following cumulative conditions: Links can only be made to webpages that provide a link to the Legal Notice page. | |
Record name | Neomycin, sulfate (salt) | |
Source | European Chemicals Agency (ECHA) | |
URL | https://echa.europa.eu/substance-information/-/substanceinfo/100.014.339 | |
Description | The European Chemicals Agency (ECHA) is an agency of the European Union which is the driving force among regulatory authorities in implementing the EU's groundbreaking chemicals legislation for the benefit of human health and the environment as well as for innovation and competitiveness. | |
Explanation | Use of the information, documents and data from the ECHA website is subject to the terms and conditions of this Legal Notice, and subject to other binding limitations provided for under applicable law, the information, documents and data made available on the ECHA website may be reproduced, distributed and/or used, totally or in part, for non-commercial purposes provided that ECHA is acknowledged as the source: "Source: European Chemicals Agency, http://echa.europa.eu/". Such acknowledgement must be included in each copy of the material. ECHA permits and encourages organisations and individuals to create links to the ECHA website under the following cumulative conditions: Links can only be made to webpages that provide a link to the Legal Notice page. | |
Q & A
Q1: How does neomycin sulfate exert its antibacterial effect?
A1: [] Neomycin sulfate is an aminoglycoside antibiotic that acts by binding to the bacterial ribosome, specifically the 30S ribosomal subunit. This interaction disrupts protein synthesis, leading to bacterial cell death. For more information on this mechanism, refer to [Formation of antibiotic, biodegradable/bioabsorbable polymers by processing with neomycin sulfate and its inclusion compound with β‐cyclodextrin].
Q2: What is the molecular formula and weight of neomycin sulfate?
A2: While the provided research papers don't explicitly state the molecular formula and weight, this information can be readily found in chemical databases like PubChem and ChemSpider. Please refer to those resources for detailed structural information.
Q3: Has neomycin sulfate been incorporated into polymeric materials for medical applications?
A3: [] Yes, neomycin sulfate has been successfully incorporated into poly(L-lactic acid) (PLLA) and poly(e-caprolactone) (PCL) films. These films demonstrated antibacterial activity against Escherichia coli, suggesting their potential use in biodegradable/bioabsorbable sutures for preventing post-surgical infections. This research is detailed in [Formation of antibiotic, biodegradable/bioabsorbable polymers by processing with neomycin sulfate and its inclusion compound with β‐cyclodextrin].
Q4: What is the impact of hexadecyl alcohol on neomycin sulfate release from polymeric films?
A4: [] Research indicates that hexadecyl alcohol can suppress or decrease the release of neomycin sulfate from both ethyl cellulose and polyamide films. Conversely, tributyl citrate, another plasticizer, was found to increase neomycin sulfate release from ethyl cellulose films. These findings highlight the importance of plasticizer selection in controlling drug release from polymeric drug delivery systems. This research is detailed in [In Vitro Release of Neomycin Sulfate from Polymeric Films].
Q5: What is the impact of pH on the stability of neomycin sulfate in solutions?
A5: [] Studies show that adjusting the pH value of neomycin sulfate solutions to between 4 and 5 using hydrochloric acid (HCl) prevents the solution from turning yellow after sterilization at 100 °C. This suggests that maintaining a slightly acidic environment enhances the stability of neomycin sulfate solutions during sterilization. This research is detailed in [Preparation and Quality Control of Sterilized Neomycin Sulfate Solution].
Q6: What is the bioavailability of neomycin sulfate after oral administration in swine?
A6: [] Research indicates that the absolute bioavailability of neomycin sulfate after oral administration in swine is relatively low at 4.84% ± 0.03. This suggests that a significant portion of the orally administered dose is not absorbed into the systemic circulation. This finding has implications for dosing regimens and efficacy of oral neomycin sulfate in swine. This research is detailed in [Pharmacokinetics of neomycin sulfate after intravenous and oral administrations in swine.].
Q7: Has neomycin sulfate been investigated for its efficacy in treating colibacillosis in turkeys?
A7: [] Yes, neomycin sulfate has been studied for its efficacy in controlling mortality associated with colibacillosis (caused by Escherichia coli) in growing turkeys. The study demonstrated that administering neomycin sulfate in drinking water effectively reduced mortality in turkeys challenged with E. coli. This research is detailed in [Efficacy of neomycin sulfate water medication on the control of mortality associated with colibacillosis in growing turkeys.].
Q8: What is the impact of neomycin sulfate on the growth of probiotic bacteria?
A8: [] Research indicates that neomycin sulfate significantly inhibits the growth of several probiotic bacteria, including Lactobacillus acidophilus, Bifidobacterium bifidum, Lactobacillus bulgaricus, Lactobacillus rhamnosus, and Lactobacillus casei. This finding suggests that neomycin sulfate use could potentially disrupt the balance of beneficial gut microbiota. This research is detailed in [Effect of polimixin B and neomycin sulfate on growth of selected probiotics].
Q9: Are there any known mechanisms of resistance to neomycin sulfate in bacteria?
A9: While the provided papers don't extensively cover specific resistance mechanisms, it is a well-known phenomenon with aminoglycosides. Resistance can develop through enzymatic inactivation of the antibiotic, modification of the ribosomal binding site, and decreased permeability of the bacterial cell wall. Understanding these mechanisms is crucial for developing strategies to combat antibiotic resistance.
Q10: What are the potential adverse effects of neomycin sulfate on the skin?
A10: Although not extensively discussed in the provided research, neomycin sulfate, like other aminoglycosides, can cause allergic contact dermatitis in some individuals. This is a type IV hypersensitivity reaction, and its symptoms can range from mild irritation to severe skin inflammation. This information highlights the importance of careful consideration and potential allergy testing before topical use.
Q11: What analytical methods are commonly used to quantify neomycin sulfate?
A11: [, , ] Several analytical methods have been employed for the quantification of neomycin sulfate, including high-performance liquid chromatography (HPLC) with pre-column derivatization and fluorescence detection, spectrophotometry, and microbiological assays. The choice of method often depends on the specific application, required sensitivity, and available resources. For more details, refer to the papers [DEVELOPMENT OF THE METHOD FOR QUANTITATIVE DETERMINATION OF NEOMYCIN SULFATE IN VETERINARY PREPARATIONS WITH PRECOLUMN DERIVATIZATION AND FLUORESCENT DETECTION], [HPLC-ELSD determination of neomycin sulfate and its related substance neamine], and [Validation of Microbiological Assay Design of Neomycin Sulfate in 30 x 30 cm Rectangular Antibiotica Plate].
Disclaimer and Information on In-Vitro Research Products
Please be aware that all articles and product information presented on BenchChem are intended solely for informational purposes. The products available for purchase on BenchChem are specifically designed for in-vitro studies, which are conducted outside of living organisms. In-vitro studies, derived from the Latin term "in glass," involve experiments performed in controlled laboratory settings using cells or tissues. It is important to note that these products are not categorized as medicines or drugs, and they have not received approval from the FDA for the prevention, treatment, or cure of any medical condition, ailment, or disease. We must emphasize that any form of bodily introduction of these products into humans or animals is strictly prohibited by law. It is essential to adhere to these guidelines to ensure compliance with legal and ethical standards in research and experimentation.