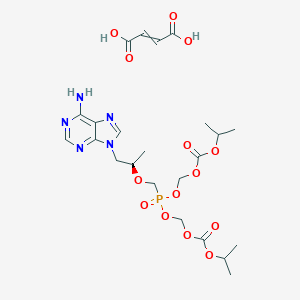
Tenofovir Disoproxil Fumarate
Overview
Description
Viread, known chemically as tenofovir disoproxil fumarate, is a medication used to treat chronic hepatitis B and to prevent and treat HIV/AIDS . It is a nucleotide reverse transcriptase inhibitor (NRTI) that works by blocking the activity of reverse transcriptase, an enzyme crucial for the replication of HIV and hepatitis B viruses .
Mechanism of Action
Target of Action
Tenofovir Disoproxil Fumarate (TDF), marketed under the trade name Viread, is a nucleotide analogue reverse transcriptase inhibitor (nRTI) . It primarily targets the HIV-1 virus and the Hepatitis B virus . The compound is used in combination with other antiretroviral agents for the treatment of HIV-1 infection and is also indicated for the treatment of chronic hepatitis B .
Mode of Action
TDF is a prodrug, which means it is metabolized into its active form, tenofovir, inside the body . Tenofovir works by inhibiting the activity of HIV-1 reverse transcriptase , an enzyme that the HIV virus needs to replicate itself . It does this by competing with the natural substrate, deoxyadenosine 5’-triphosphate, and causing DNA chain termination .
Biochemical Pathways
TDF affects several biochemical pathways. It has been found to induce neuronal cell apoptosis through increasing the intracellular reactive oxygen species (ROS) and the expression level of caspase-3 . This suggests that TDF can ameliorate chronic liver diseases (CLDs) independently of its antiviral activity by affecting the expression of genes involved in hepatic immune, inflammatory, and metabolic processes .
Pharmacokinetics
TDF has a bioavailability of 25% . It is metabolized through ester hydrolysis into its active form, tenofovir . As tenofovir is eliminated by the kidney, the coadministration of other drugs that reduce renal activity or compete for renal elimination may result in increased serum concentrations of either tenofovir or the coadministered drug .
Result of Action
The primary result of TDF’s action is the inhibition of viral replication, which helps in the management of HIV-1 infection and the treatment of Hepatitis B . . For instance, it has been found to induce apoptosis in neuronal cells . It also affects the expression of genes involved in hepatic immune, inflammatory, and metabolic processes .
Action Environment
Environmental factors can influence the action, efficacy, and stability of TDF. For instance, lower weight and lower BMI have been found to be key risk factors for TDF-induced nephrotoxicity . This is because reduced plasma TDF clearance among people with lower body weight leads to larger drug exposure and higher plasma concentrations of TDF, resulting in more severe toxicity and renal tubular dysfunction . Furthermore, TDF has been detected in surface water, indicating that it can persist in the environment .
Biochemical Analysis
Biochemical Properties
Tenofovir Disoproxil Fumarate is a prodrug of tenofovir . Once inside the target cells, it is converted to its active form, tenofovir, a nucleoside monophosphate (nucleotide) analog . This conversion involves the hydrolysis of the disoproxil moiety .
Cellular Effects
This compound has been shown to affect neurogenesis in the brains of mice and mitochondria in neurons . It has also been observed to cause peripheral neuropathy in mice and alterations in inflammatory signaling and mitochondrial activity in the brain .
Molecular Mechanism
The primary mechanism of action of this compound involves its conversion to tenofovir through the hydrolysis of the disoproxil moiety once the compound enters the target cells . Tenofovir is then phosphorylated to tenofovir diphosphate by cellular kinases . This molecule inhibits the pursuit of HIV-1 reverse transcriptase – a mechanism which HIV-1 virus uses to replicate – by blocking its action .
Temporal Effects in Laboratory Settings
Chronic administration of this compound to adult Wistar rats resulted in proximal tubular damage, proximal tubular dysfunction, and extensive proximal tubular mitochondrial injury . A significant decline in mean liver stiffness between baseline and last visit was observed in this compound treated rats .
Dosage Effects in Animal Models
In animal models, this compound has been shown to induce peripheral neuropathy and alter inflammation and mitochondrial biogenesis in the brains of mice . It has also been observed to cause a significant reduction in sperm count and motility, ultimately affecting male fertility .
Metabolic Pathways
This compound modulates lipid metabolism by upregulating hepatic CD36 via PPAR-α activation . Dyslipidemia could be associated with arteriosclerosis and hepatocarcinogenesis .
Transport and Distribution
The volume of distribution at steady-state for this compound is 1.3 ± 0.6 L/kg and 1.2 ± 0.4 L/kg, following intravenous administration of tenofovir 1.0 mg/kg and 3.0 mg/kg respectively .
Subcellular Localization
This compound is predominantly eliminated via the proximal tubules of the kidney . Damaged mitochondria are major sources of reactive oxygen species and cellular damage is reported to occur after the antioxidants are depleted .
Preparation Methods
Synthetic Routes and Reaction Conditions
The synthesis of tenofovir disoproxil fumarate involves multiple steps, starting from the precursor tenofovir. The process includes esterification reactions to form the disoproxil ester, followed by fumarate salt formation . The reaction conditions typically involve the use of organic solvents and catalysts to facilitate the esterification and salt formation processes.
Industrial Production Methods
Industrial production of this compound follows similar synthetic routes but on a larger scale. The process is optimized for high yield and purity, involving stringent quality control measures to ensure the final product meets pharmaceutical standards .
Chemical Reactions Analysis
Types of Reactions
Tenofovir disoproxil fumarate undergoes various chemical reactions, including hydrolysis, oxidation, and reduction .
Common Reagents and Conditions
Oxidation and Reduction: These reactions involve the use of oxidizing or reducing agents to modify the chemical structure of this compound.
Major Products Formed
The primary product formed from the hydrolysis of this compound is tenofovir, which is the active form of the drug .
Scientific Research Applications
Tenofovir disoproxil fumarate has a wide range of scientific research applications:
Chemistry: It is used as a model compound in studies involving nucleotide analogs and their interactions with enzymes.
Medicine: It is extensively studied for its efficacy in treating HIV and hepatitis B infections.
Industry: The compound is used in the development of new antiviral drugs and formulations.
Comparison with Similar Compounds
Similar Compounds
Tenofovir alafenamide: Another prodrug of tenofovir, known for its improved renal and bone safety profile compared to tenofovir disoproxil fumarate.
Emtricitabine: Often used in combination with this compound for enhanced antiviral efficacy.
Bictegravir: Combined with tenofovir alafenamide and emtricitabine in a single tablet for the treatment of HIV.
Uniqueness
This compound is unique due to its high efficacy in suppressing viral replication and its extensive use in both HIV and hepatitis B treatments . Its ability to be used in combination with other antiviral agents further enhances its therapeutic potential .
Properties
CAS No. |
202138-50-9 |
---|---|
Molecular Formula |
C23H34N5O14P |
Molecular Weight |
635.5 g/mol |
IUPAC Name |
[[(2R)-1-(6-aminopurin-9-yl)propan-2-yl]oxymethyl-(propan-2-yloxycarbonyloxymethoxy)phosphoryl]oxymethyl propan-2-yl carbonate;but-2-enedioic acid |
InChI |
InChI=1S/C19H30N5O10P.C4H4O4/c1-12(2)33-18(25)28-9-31-35(27,32-10-29-19(26)34-13(3)4)11-30-14(5)6-24-8-23-15-16(20)21-7-22-17(15)24;5-3(6)1-2-4(7)8/h7-8,12-14H,6,9-11H2,1-5H3,(H2,20,21,22);1-2H,(H,5,6)(H,7,8)/t14-;/m1./s1 |
InChI Key |
VCMJCVGFSROFHV-PFEQFJNWSA-N |
Isomeric SMILES |
C[C@H](CN1C=NC2=C(N=CN=C21)N)OCP(=O)(OCOC(=O)OC(C)C)OCOC(=O)OC(C)C.C(=CC(=O)O)C(=O)O |
SMILES |
CC(C)OC(=O)OCOP(=O)(COC(C)CN1C=NC2=C(N=CN=C21)N)OCOC(=O)OC(C)C.C(=CC(=O)O)C(=O)O |
Canonical SMILES |
CC(C)OC(=O)OCOP(=O)(COC(C)CN1C=NC2=C(N=CN=C21)N)OCOC(=O)OC(C)C.C(=CC(=O)O)C(=O)O |
Appearance |
Assay:≥98%A crystalline solid |
Color/Form |
White, fine, powder-like crystals |
melting_point |
113-115 |
202138-50-9 | |
Pictograms |
Corrosive; Irritant; Health Hazard |
solubility |
In water, 13.4 mg/mL at 25 °C |
Synonyms |
(R)-9-(2-phosphonylmethoxypropyl)adenine 9-(2-phosphonomethoxypropyl)adenine 9-(2-phosphonylmethoxypropyl)adenine 9-(2-phosphonylmethoxypropyl)adenine, (+-)-isomer 9-(2-Phosphonylmethoxypropyl)adenine, (R)-isomer - T357098 9-(2-phosphonylmethoxypropyl)adenine, (S)-isomer 9-PMPA (tenofovir) Disoproxil Fumarate, Tenofovir Disoproxil, Tenofovir Fumarate, Tenofovir Disoproxil tenofovir tenofovir disoproxil tenofovir disoproxil fumarate Viread |
Origin of Product |
United States |
Retrosynthesis Analysis
AI-Powered Synthesis Planning: Our tool employs the Template_relevance Pistachio, Template_relevance Bkms_metabolic, Template_relevance Pistachio_ringbreaker, Template_relevance Reaxys, Template_relevance Reaxys_biocatalysis model, leveraging a vast database of chemical reactions to predict feasible synthetic routes.
One-Step Synthesis Focus: Specifically designed for one-step synthesis, it provides concise and direct routes for your target compounds, streamlining the synthesis process.
Accurate Predictions: Utilizing the extensive PISTACHIO, BKMS_METABOLIC, PISTACHIO_RINGBREAKER, REAXYS, REAXYS_BIOCATALYSIS database, our tool offers high-accuracy predictions, reflecting the latest in chemical research and data.
Strategy Settings
Precursor scoring | Relevance Heuristic |
---|---|
Min. plausibility | 0.01 |
Model | Template_relevance |
Template Set | Pistachio/Bkms_metabolic/Pistachio_ringbreaker/Reaxys/Reaxys_biocatalysis |
Top-N result to add to graph | 6 |
Feasible Synthetic Routes
A: Viread, a prodrug of tenofovir, exerts its antiviral effect by inhibiting viral DNA polymerase. [] Once inside the cell, Viread is metabolized to tenofovir, which is then phosphorylated to its active metabolite, tenofovir diphosphate. Tenofovir diphosphate acts as an analog of adenosine 5'-triphosphate and competes with it for incorporation into viral DNA. Once incorporated, it causes chain termination, halting viral DNA synthesis. []
A: By inhibiting viral DNA polymerase, Viread effectively blocks the conversion of viral RNA to DNA. [, ] This inhibition has a cascading effect, preventing the integration of viral DNA into the host cell genome and ultimately halting the production of new viral particles. [, ]
A: The molecular formula of Tenofovir Disoproxil Fumarate is C20H30N5O10P • C4H4O4, and its molecular weight is 635.5 g/mol. []
A: Tenofovir disoproxil is susceptible to hydrolysis, potentially limiting its absorption in the intestinal tract. [] To improve stability, formulations often use non-hygroscopic excipients and stabilizers like sodium bisulfite. []
A: Tenofovir, an acyclic nucleoside phosphonate, mimics natural nucleosides, allowing it to interact with viral DNA polymerase. [, ] The phosphonate group is crucial for its activity as it bypasses the first phosphorylation step required for activation. [, ]
A: Yes, modifications like the addition of a hexadecyloxypropyl group to tenofovir (CMX157) have demonstrated significantly increased antiviral activity compared to tenofovir. [] This is attributed to enhanced cellular uptake and higher intracellular levels of the active metabolite. []
A: Several approaches have been investigated, including the development of stabilized tablets using non-hygroscopic excipients. [] One notable strategy involves esterification with lipids, creating lysolecithin mimics like CMX157, which utilizes natural uptake pathways for improved oral bioavailability. [] Additionally, 3D-printed dose-flexible printlets of this compound have been developed using selective laser sintering, demonstrating bioequivalence to commercial tablets. []
A: Viread is administered orally and is rapidly absorbed. [] It undergoes ester hydrolysis to form tenofovir, which is then further phosphorylated to its active metabolite, tenofovir diphosphate, primarily within lymphocytes and other target cells. [, ]
A: Studies comparing Viread to its newer formulation, tenofovir alafenamide (TAF), suggest differences in tissue distribution. TAF achieves higher concentrations in target tissues, including the lymphatic system, while exhibiting lower concentrations in the blood. []
A: Yes, Viread has shown efficacy in treating HIV-1 infection in both treatment-naïve and treatment-experienced adult patients. [, ] It has also shown efficacy in treating chronic hepatitis B infection in adults and adolescents. [, , ]
A: Animal studies have provided data supporting the potential of using Viread for pre-exposure prophylaxis (PrEP) to prevent HIV infection. []
A: Resistance to Viread is primarily associated with mutations in the HIV reverse transcriptase gene, specifically the K65R mutation. [] This mutation reduces the drug's ability to bind to the enzyme and inhibit its activity. []
A: Cross-resistance with other nucleoside/nucleotide reverse transcriptase inhibitors (NRTIs) is a concern, particularly with drugs like didanosine and lamivudine. The emergence of the K65R mutation can confer resistance to multiple drugs in this class. []
A: While generally well-tolerated, long-term use of Viread has been associated with renal dysfunction, including decreased glomerular filtration rate and, in some cases, Fanconi syndrome. [, ]
A: Some studies have reported a small but statistically significant decrease in bone mineral density in individuals taking Viread, emphasizing the need for monitoring bone health in long-term users. []
A: Monitoring serum creatinine levels is crucial in assessing renal function in patients on Viread. [, ] Additionally, monitoring for proteinuria and other markers of tubular dysfunction can aid in the early detection of potential renal toxicity. [, ]
A: Tenofovir alafenamide (TAF) is a newer prodrug of tenofovir with a more favorable safety profile, particularly regarding renal and bone health. [, ] TAF achieves higher intracellular drug concentrations in target cells with lower plasma concentrations, potentially reducing systemic exposure and toxicity. [, ]
A: The United States Food and Drug Administration (FDA) approved Viread for the treatment of HIV-1 infection in October 2001. []
A: One major development has been the introduction of tenofovir alafenamide (TAF), a newer prodrug with a potentially improved safety profile. [, ] Additionally, the development of fixed-dose combinations containing Viread, such as Truvada (emtricitabine/tenofovir disoproxil fumarate) and Atripla (efavirenz/emtricitabine/tenofovir disoproxil fumarate), has simplified treatment regimens and improved adherence. [, ]
Disclaimer and Information on In-Vitro Research Products
Please be aware that all articles and product information presented on BenchChem are intended solely for informational purposes. The products available for purchase on BenchChem are specifically designed for in-vitro studies, which are conducted outside of living organisms. In-vitro studies, derived from the Latin term "in glass," involve experiments performed in controlled laboratory settings using cells or tissues. It is important to note that these products are not categorized as medicines or drugs, and they have not received approval from the FDA for the prevention, treatment, or cure of any medical condition, ailment, or disease. We must emphasize that any form of bodily introduction of these products into humans or animals is strictly prohibited by law. It is essential to adhere to these guidelines to ensure compliance with legal and ethical standards in research and experimentation.