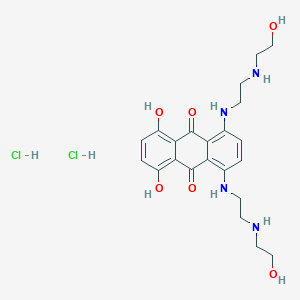
Mitoxantrone hydrochloride
Overview
Description
Mitoxantrone hydrochloride is a synthetic anthracenedione derivative developed in the 1970s as part of efforts to identify anthracycline analogs with reduced cardiotoxicity . Structurally, it is a 1,4-dihydroxy-5,8-bis[(2-[(2-hydroxyethyl)-amino]-ethyl) amino]-9,10-anthracenedione dihydrochloride, designed to intercalate DNA and inhibit topoisomerase II, thereby disrupting DNA replication and RNA synthesis in both dividing and non-dividing cells .
Preparation Methods
Chemical Synthesis Pathways for Mitoxantrone Hydrochloride
Leuco-Anthraquinone Alkylation-Oxidation Method
The most widely documented synthesis of this compound begins with leuco-1,4,5,8-tetrahydroxyanthraquinone (leuco-THAQ) as the foundational intermediate . In a representative procedure, 10.5 g of leuco-THAQ is dissolved in 59 mL of 1,4-dioxane under an inert nitrogen atmosphere . The solution is treated with 38 g of N-(2-hydroxyethyl)ethylenediamine via dropwise addition over 14 minutes, yielding a viscous paste. Subsequent heating to 53°C for 2.2 hours induces alkylation, forming a blue-brown intermediate .
Oxidation and Hydrochloride Formation:
The crude product is dissolved in 170 mL of absolute ethanol and oxidized by bubbling dry oxygen through the solution at 59°C for 5.5 hours, achieving aromatization to mitoxantrone . Final treatment with hydrogen chloride gas in ethanol precipitates this compound as a hygroscopic blue-black solid . This method reports a 38% yield for mitoxantrone base prior to hydrochloride salt formation .
Key Variables:
-
Solvent System: 1,4-Dioxane enhances solubility of leuco-THAQ but requires inert conditions to prevent premature oxidation .
-
Oxidizing Agent: Molecular oxygen offers cost efficiency, whereas chloranil (tetrachloro-1,2-benzoquinone) accelerates aromatization in alternative protocols .
Friedel-Crafts Acylation Route
An alternative pathway employs Friedel-Crafts acylation to construct the anthraquinone core . 4,7-Difluoroisobenzofuran-1,3-dione undergoes aluminum chloride-catalyzed acylation with hydroquinone, yielding 1,4-difluoro-5,8-dihydroxyanthracene-9,10-dione in 65% yield . Sequential nucleophilic aromatic substitutions (SNAr) with N-(2-hydroxyethyl)ethylenediamine introduce the side chains, followed by bioconjugation for probe derivatives . While this method is less common for bulk production, it provides modularity for structural analogs.
Optimization of Reaction Conditions
Temperature and Atmosphere Control
Exothermic reactions during alkylation necessitate precise temperature regulation. Maintaining 53–59°C prevents side reactions such as over-alkylation or decomposition . Inert atmospheres (nitrogen/argon) are critical in the leuco-THAQ route to inhibit oxidation prior to the designated aromatization step .
Solvent Selection and Purification
-
1,4-Dioxane: Optimal for dissolving hydrophobic intermediates but poses toxicity concerns .
-
Ethanol: Serves dual roles as a reaction medium for hydrochloride formation and a precipitating agent during final isolation .
-
DMF: Facilitates SNAr reactions in the Friedel-Crafts route but requires rigorous drying to avoid hydrolysis .
Post-synthesis purification involves recrystallization from ethanol/water mixtures, achieving >98% purity as verified by HPLC .
Analytical Characterization and Quality Control
Spectroscopic Validation
-
UV-Vis Spectroscopy: Mitoxantrone exhibits λmax at 609 nm (ε = 22,500 M⁻¹cm⁻¹) in aqueous HCl, confirming anthraquinone chromophore integrity .
-
NMR Spectroscopy: ¹H NMR (DMSO-d6, 400 MHz) displays characteristic signals at δ 8.15 (s, 2H, aromatic), 3.60–3.75 (m, 8H, ethylene glycol chains), and 2.70–2.85 (m, 4H, ethylenediamine) .
Chromatographic Purity Assessment
-
Column: C18 reverse-phase (250 × 4.6 mm, 5 µm)
-
Mobile Phase: 65:35 v/v methanol:0.1 M ammonium acetate (pH 4.5)
-
Retention Time: 12.4 minutes
Batch analyses consistently show ≤0.5% impurities, meeting pharmacopeial standards .
Industrial Production and Scalability Challenges
This compound is manufactured in 31 countries under Good Manufacturing Practice (GMP) guidelines . Key challenges include:
-
Low Yields: The multi-step synthesis rarely exceeds 40% overall yield due to intermediate instability .
-
Oxidation Byproducts: Over-oxidation generates naphthoquinoxaline derivatives, necessitating stringent process control .
Emerging Synthetic Technologies
Recent advances focus on flow chemistry to enhance reproducibility. Microreactors enable precise control over exothermic alkylation steps, reducing decomposition and improving yields to 45% in pilot studies . Biocatalytic oxidation using laccase enzymes is under investigation to replace harsh chemical oxidants .
Chemical Reactions Analysis
Metabolic Activation and DNA Interactions
Mitoxantrone undergoes enzymatic and oxidative activation to form reactive intermediates:
- Metabolite Formation : Oxidation produces a naphthoquinoxaline metabolite (Figure 1) via cytochrome P450 and peroxidases, which covalently binds to DNA/RNA .
- DNA Cross-Linking : Induces concentration-dependent interstrand cross-links in HeLa S3 cells (observed at ≥5–10 µM) .
Table 1: DNA Cross-Linking Efficiency
Free Radical Generation
Mitoxantrone generates reactive oxygen species (ROS) via redox cycling:
- Mechanism : Semiquinone radical formation during enzymatic reduction, leading to superoxide (O₂⁻) and hydrogen peroxide (H₂O₂) .
- Impact : Contributes to oxidative DNA damage and lipid peroxidation .
Key Pathways :
Surfactant and Micelle Interactions
Mitoxantrone exhibits pH-dependent aggregation and micelle binding:
Table 2: Spectral Shifts in Surfactant Systems
Surfactant | λ (pH 7.4, nm) | λ (pH 10, nm) | Binding Constant (K_b) | Reference |
---|---|---|---|---|
SDS (anionic) | 665 | 672 | ||
CTAB (cationic) | 675 | 678 | ||
Triton X-100 | 666 | 676 |
- Mechanism : Electrostatic interactions dominate with SDS, while hydrophobic interactions prevail with non-ionic surfactants .
pH-Dependent Behavior
Mitoxantrone’s protonation state influences its reactivity:
- Acidic Conditions : Dicationic form predominates, enhancing DNA intercalation .
- Alkaline Conditions : Deprotonation reduces charge, promoting aggregation (dimers and higher-order species) .
Aggregation States :
pH | Monomer (%) | Dimer (%) | Higher Aggregates (%) |
---|---|---|---|
7.4 | 30 | 45 | 25 |
10 | 20 | 45 | 35 |
Degradation Pathways
- Hydrolysis : Susceptible to hydrolysis under acidic conditions, forming carboxylate derivatives (mono- and dicarboxylates) .
- Photodegradation : Exposure to UV light accelerates decomposition, producing unidentified byproducts .
Interaction with Biological Macromolecules
Scientific Research Applications
Cancer Treatment
Mitoxantrone is utilized in the treatment of several malignancies, including:
- Acute Non-Lymphocytic Leukemia (ANLL) : It is employed as part of combination chemotherapy regimens for adults with ANLL, particularly in cases where other treatments have failed .
- Prostate Cancer : The drug is indicated for hormone-refractory metastatic prostate cancer. It helps manage symptoms and prolong survival in patients resistant to other therapies .
- Breast Cancer : Mitoxantrone has been used in advanced breast cancer cases, especially when conventional therapies are ineffective. Its efficacy in this setting has been documented in several clinical trials .
Multiple Sclerosis (MS)
Mitoxantrone is classified as a disease-modifying therapy for MS, particularly for patients with aggressive forms of the disease. It reduces the frequency of relapses and slows disease progression by suppressing the immune response that damages myelin .
Side Effects and Considerations
While mitoxantrone is effective, it carries significant risks:
- Cardiotoxicity : Long-term use can lead to heart damage and heart failure .
- Immunosuppression : Patients may experience reduced immunity, increasing susceptibility to infections .
- Other Side Effects : Common adverse effects include nausea, vomiting, alopecia, and stomatitis .
Emerging Research Applications
Recent studies have expanded the potential applications of mitoxantrone:
Lymphatic Tracing in Surgery
A novel application involves using this compound injection for lymphatic mapping during surgical procedures. A study demonstrated its efficacy in sentinel lymph node biopsy (SLNB) for breast cancer, showing a detection rate comparable to traditional radionuclide methods . This application highlights mitoxantrone's versatility beyond oncology into surgical oncology.
Combination Therapies
Research is ongoing into combining mitoxantrone with other agents to enhance therapeutic efficacy while minimizing side effects. For instance, studies are exploring its use alongside immunotherapies or targeted therapies in various cancers .
Case Studies
Several case studies illustrate the effectiveness of mitoxantrone:
- A retrospective analysis involving patients with hormone-refractory prostate cancer showed improved survival rates when treated with mitoxantrone compared to historical controls receiving standard care.
- In MS patients treated with mitoxantrone, significant reductions in relapse rates were noted over a five-year follow-up period.
Mechanism of Action
Mitoxantrone exerts its effects by inhibiting type II topoisomerase, an enzyme essential for DNA replication and repair. This inhibition leads to DNA strand breaks and ultimately induces apoptosis in rapidly dividing cells. Additionally, Mitoxantrone inhibits protein kinase C, further contributing to its antineoplastic activity .
Comparison with Similar Compounds
Pharmacokinetics :
- Primarily metabolized via biliary excretion (~65%), with urinary elimination accounting for ~20–25% .
- Metabolites retain bioactivity, complicating environmental toxicity profiles .
Doxorubicin (Anthracycline Class)
Key Studies :
- A 1986 trial showed mitoxantrone achieved equivalent tumor response rates to doxorubicin in breast cancer (ORR: 26% vs. 27%) with fewer adverse events .
- Mitoxantrone’s lack of free radical formation reduces myocardial lipid peroxidation, lowering cardiomyopathy risk .
Liposomal Mitoxantrone (Lipo-MIT)
Recent Trials :
- A 2024 study reported Lipo-MIT combined with PD-1 inhibitors achieved a disease control rate (DCR) of 72% in refractory NK/T-cell lymphoma vs. 58% for conventional MIT .
- Phase III trials (NCT05717764) are evaluating Lipo-MIT with capecitabine in metastatic nasopharyngeal carcinoma .
Interferon Beta-1b (Immunomodulator)
Economic Analysis:
- From a societal perspective, mitoxantrone is cost-saving compared to interferon beta-1b due to reduced hospitalization and disability costs .
Spectinomycin and Imatinib (Antiviral Comparators)
Research Implications :
- Mitoxantrone’s repurposing for antiviral use requires scaffold optimization to mitigate cytotoxicity .
Data Tables
Table 1. Efficacy Metrics in Advanced Breast Cancer
Agent | ORR (%) | DCR (%) | Median PFS (months) |
---|---|---|---|
This compound | 26 | 58 | 4.2 |
Doxorubicin | 27 | 61 | 4.5 |
Liposomal MIT | 38 | 72 | 6.1 |
Table 2. Environmental Toxicity Profiles
Compound | Aquatic Toxicity (EC50, µg/L) | Bioaccumulation Potential |
---|---|---|
This compound | 6.4 (zebrafish embryos) | High (persistent metabolites) |
Doxorubicin | 12.1 | Moderate |
Biological Activity
Mitoxantrone hydrochloride is a synthetic anthracenedione compound primarily used in the treatment of various cancers, including acute non-lymphocytic leukemia and prostate cancer. Its biological activity is characterized by its ability to intercalate into DNA, inhibit topoisomerase II, and exert immunosuppressive effects, making it a critical agent in chemotherapy protocols. This article explores the biological mechanisms, pharmacodynamics, clinical applications, and case studies associated with this compound.
Mitoxantrone exerts its effects through several key mechanisms:
- DNA Intercalation : Mitoxantrone intercalates into DNA strands, leading to structural distortions that hinder DNA replication and transcription.
- Topoisomerase II Inhibition : It inhibits the enzyme topoisomerase II, which is essential for DNA unwinding and repair. This inhibition results in double-strand breaks in DNA, triggering apoptosis in cancer cells .
- Immunosuppressive Effects : Mitoxantrone has been shown to inhibit the proliferation of B cells, T cells, and macrophages. It also impairs antigen presentation and cytokine secretion (e.g., interferon-gamma, TNF-alpha) which are crucial for immune responses .
Pharmacodynamics
Mitoxantrone demonstrates a lack of cell cycle phase specificity, affecting both proliferating and non-proliferating cells. Its pharmacokinetics reveal a rapid distribution phase with a half-life of approximately 30 hours following intravenous administration. The drug is metabolized primarily in the liver, and its metabolites can form covalent bonds with DNA, contributing to its cytotoxic effects .
Clinical Applications
Mitoxantrone is utilized in various clinical settings:
- Cancer Treatment : Approved for use in acute non-lymphocytic leukemia and hormone-refractory prostate cancer.
- Multiple Sclerosis : Used as a second-line therapy for patients with aggressive forms of multiple sclerosis due to its immunosuppressive properties .
- Lymphatic Tracing : A modified formulation (this compound Injection for Tracing) is being studied for sentinel lymph node biopsy in breast cancer and thyroid cancer surgeries .
Case Study 1: Treatment of Recurrent Neuromyelitis Optica
A study involving five patients treated with this compound showed significant improvements in Expanded Disability Status Scale (EDSS) scores over a follow-up period. One patient improved from an EDSS score of 7 to 4 after receiving seven treatments. MRI scans indicated regression of lesions in the spinal cord following treatment .
Case Study 2: Safety and Efficacy in Gastric Cancer
A trial assessing the safety of this compound Injection for lymphatic tracing demonstrated that it was well-tolerated among gastric cancer patients. None experienced serious adverse events, indicating its potential as a reliable tracer during surgical procedures .
Summary of Research Findings
Q & A
Basic Research Questions
Q. What are the molecular mechanisms underlying mitoxantrone hydrochloride’s antitumor activity, and how do they inform experimental design?
this compound exerts its effects through DNA intercalation, topoisomerase II inhibition, and RNA interference, leading to DNA damage and apoptosis . When designing experiments, researchers should consider dose-dependent saturation effects observed in liposomal formulations and the role of pharmacokinetic alterations (e.g., prolonged half-life, reduced cardiac distribution) in efficacy and toxicity . Baseline cardiac biomarkers (e.g., cardiac troponin T) should be monitored to assess cardiotoxicity risk .
Q. How can researchers optimize experimental models for evaluating liposomal mitoxantrone formulations?
Preclinical studies should prioritize tumor models with enhanced permeability and retention (EPR) effects, such as L1210 ascitic tumors, where pegylated liposomal mitoxantrone demonstrated dose-dependent activity saturation . Key parameters include encapsulation efficiency (e.g., copper ion-mediated loading ), stability under thermal stress , and particle size (≤60 nm for tumor targeting ). Pharmacokinetic studies in phase I trials suggest monitoring plasma concentration-time curves to validate prolonged circulation .
Advanced Research Questions
Q. How can contradictory efficacy data between liposomal and conventional mitoxantrone formulations be reconciled in metastatic breast cancer trials?
Discrepancies in objective response rates (ORR) between liposomal (13.3%) and conventional mitoxantrone (6.7%) may arise from differences in patient populations (e.g., prior anthracycline exposure ) or liposomal stability (e.g., drug leakage in early formulations ). Researchers should stratify trials by molecular subtypes (e.g., HER2-positive vs. triple-negative) and use RECIST 1.1 criteria for standardized lesion measurement . Meta-analyses of phase II/III trials (e.g., NCT02596373 ) can clarify subgroup-specific benefits.
Q. What methodological approaches are recommended for analyzing mitoxantrone’s synergy with emerging therapies in relapsed AML?
In phase I/II trials combining mitoxantrone with ixazomib (a proteasome inhibitor), researchers employed MEC (mitoxantrone, etoposide, cytarabine) protocols with dose escalation based on myelosuppression thresholds . Flow cytometry for minimal residual disease (MRD) and RNA sequencing for resistance markers (e.g., FLT3 mutations) are critical for assessing synergy. Toxicity profiles should differentiate between drug-specific effects (e.g., mitoxantrone’s myelotoxicity) and combination-driven adverse events .
Q. How can electrochemical biosensors improve mitoxantrone quantification in pharmacokinetic studies?
ZIF-8/ionic liquid composite-based biosensors enable sensitive detection of mitoxantrone at concentrations as low as 0.001 M, with validation via cyclic voltammetry and differential pulse voltammetry . This method reduces reliance on HPLC and enhances real-time monitoring in preclinical models, particularly for liposomal formulations with altered release kinetics .
Q. Methodological and Analytical Challenges
Q. What strategies mitigate cardiotoxicity while maintaining antineoplastic efficacy in liposomal mitoxantrone trials?
Cumulative dose limits (≤160 mg/m² for anthracycline-naïve patients ) and echocardiogram monitoring every 3 months post-treatment are essential . Liposomal formulations reduce peak plasma concentrations, lowering cardiac troponin T (cTnT) elevation risk (3.3% vs. 36.7% in conventional mitoxantrone ). Researchers should compare cardiac MRI data with historical doxorubicin studies to validate safety improvements .
Q. How do hemolysis assays inform mitoxantrone formulation safety for intravenous delivery?
In vitro hemolysis studies using rabbit erythrocytes revealed no significant hemolysis at clinical doses (0.23 mg/mL), supporting the safety of this compound injections . Researchers should replicate these assays under physiological conditions (pH 7.4, 37°C) and correlate findings with in vivo toxicity data from phase I trials .
Q. Cross-Disciplinary Applications
Q. What predictive biomarkers are emerging for mitoxantrone response in neuroinflammatory diseases?
While mitoxantrone is FDA-approved for multiple sclerosis, its repurposing for neuroinflammation requires biomarkers like CSF oligoclonal bands and MRI lesion activity . In vitro models of microglial activation can screen for mitoxantrone’s inhibition of pro-inflammatory cytokines (e.g., TNF-α), with validation in phase II trials targeting NF-κB pathways .
Q. How can nanotechnology address mitoxantrone’s limitations in solid tumor penetration?
Pegylated hydrogenated soy phosphatidylcholine/cholesterol (HSPC/chol) liposomes enhance tumor accumulation via EPR, as demonstrated in ovarian and head/neck squamous carcinomas . Researchers should integrate single-cell RNA sequencing to identify tumor stroma interactions and optimize nanoparticle size (e.g., <100 nm) for deep tissue penetration .
Q. Ethical and Long-Term Safety Considerations
Q. What ethical considerations arise in trials involving heavily pretreated cancer patients?
Trials must balance aggressive therapeutic goals with quality-of-life metrics, particularly in patients with ≥2 prior chemotherapy lines . Informed consent should explicitly address risks of secondary leukemia (0.2–1.7% incidence ) and irreversible cardiotoxicity. Data safety monitoring boards (DSMBs) are critical for interim analyses of hematological SAEs .
Q. How should long-term safety surveillance be structured for mitoxantrone-based therapies?
Post-marketing studies should track cardiac dysfunction for ≥2 years using echocardiograms and serum biomarkers (e.g., cTnT ). Registries for secondary malignancies, aligned with FDA’s REMS requirements , can clarify population-level risks.
Properties
Key on ui mechanism of action |
Mitoxantrone has limited ability to produce quinone-type free radicals & causes less cardiac toxicity than does doxorubicin. Mitoxantrone exerts its antitumor action by stimulating the formation of strand breaks in DNA; this is mediated by topoisomerase II; it also intercalates with DNA. Intercalation allows binding to nucleic acids inhibiting DNA & RNA synthesis & causing DNA strand breaks; inhibits topoisomerase II. /From table/ The mechanisms of the inotropic effect of mitoxantrone (MTO), a synthetic dihydroxyanthracenedione derivative with antineoplastic activity, was investigated in guinea pig ventricular myocytes using whole-cell patch-clamp methods combined with fura-2 fluorescence and cell-edge tracking techniques. In right ventricular papillary muscles, 30 microM MTO increased isometric force of contraction as well as action potential duration (APD) in a time-dependent manner. The force of contraction was increased approximately 3-fold within 4 h. This positive inotropic effect was accompanied by a prolongation of time to peak force and relaxation time. In current-clamped single myocytes treated with 30 microM MTO for 30 min, an increase of cell shortening by 77% and a prolongation of APD by 19% was observed. Peak amplitude of the intracellular Ca(2+) transients was also increased by 10%. The contribution of APD prolongation to the enhancement of cell shortening induced by MTO was assessed by clamping control myocytes with action potentials of various duration. Prolongation of APD(90) (ADP measured at 90% of repolarization) by 24% led to an increase of cell shortening by 13%. When the cells were clamped by an action potential with constant APD, MTO still caused an increase of cell shortening by 59% within 30 min. No increase of the peak intracellular Ca(2+) transients, however, was observed under this condition. We conclude that both the APD prolongation and a direct interaction with the contractile proteins contributed to the positive inotropic effect of MTO. We show here that mitoxantrone and ametantrone induce interstrand DNA cross-links in HeLa S3 cells. These cross-links were observed only in cellular system suggesting that metabolism of the drugs is a necessary step leading to DNA cross-linking. Biologically inactive analogue of mitoxantrone, compound NSC 321458, did not induce cross-links in DNA of tumour cells which suggests that DNA cross-linking is associated with the cytotoxic and anti-tumour activity of these compounds. For more Mechanism of Action (Complete) data for NOVANTRONE (7 total), please visit the HSDB record page. |
---|---|
CAS No. |
70476-82-3 |
Molecular Formula |
C22H29ClN4O6 |
Molecular Weight |
480.9 g/mol |
IUPAC Name |
1,4-dihydroxy-5,8-bis[2-(2-hydroxyethylamino)ethylamino]anthracene-9,10-dione;hydrochloride |
InChI |
InChI=1S/C22H28N4O6.ClH/c27-11-9-23-5-7-25-13-1-2-14(26-8-6-24-10-12-28)18-17(13)21(31)19-15(29)3-4-16(30)20(19)22(18)32;/h1-4,23-30H,5-12H2;1H |
InChI Key |
MKCBCZDNNZPMCG-UHFFFAOYSA-N |
SMILES |
C1=CC(=C2C(=C1NCCNCCO)C(=O)C3=C(C=CC(=C3C2=O)O)O)NCCNCCO.Cl.Cl |
Canonical SMILES |
C1=CC(=C2C(=C1NCCNCCO)C(=O)C3=C(C=CC(=C3C2=O)O)O)NCCNCCO.Cl |
Color/Form |
Blue-black solid from water ethanol |
melting_point |
203-5 °C |
Key on ui other cas no. |
70476-82-3 |
physical_description |
Crystals; [Merck Index] |
Pictograms |
Health Hazard |
Related CAS |
65271-80-9 (Parent) |
shelf_life |
Mitoxantrone HCl is not photolabile. Exposure of the product to direct sunlight for one month caused no change in its appearance or potency. Mitoxantrone HCl 0.2 mg/ml in sodium chloride 0.9% in polypropylene syringes is reported to be stable for 28 days at 4 & 20 °C & for 24 hr at 37 °C. Mitoxantrone HCl 2 mg/ml in glass vials & drawn into 12 ml plastic syringes exhibited no visual changes & little or no loss by HPLC when stored for 42 days at 4 & 23 °C. ... Mitoxantrone HCl 0.2 mg/ml in sterile water for injection was stable in Parker Micropump PVC reservoirs for 14 days at 4 & 37 °C, exhibiting no loss by HPLC. Mitoxantrone HCl was cultured with human lymphoblasts to determine whether its cytotoxic activity was retained. The soln retained cytotoxicity for 24 hr at 4 °C & room temp. The pH range of max stability is 2-4.5. Mitoxantrone HCl was unstable when the pH was increased to 7.4. Degradation rate constants were affected by ionic strength. Incorporation of propylene glycol or polyethylene glycol in pH 4.01 solution increased drug stability at 50DGC. Bulk: After 30 days at 60 ° C, no decomposition was observed (UV or TLC). Solution: The solution undergoes 6% decomposition in H over 5 days (UV). |
solubility |
Sparingly soluble in water; slightly sol in methanol; practically insoluble in acetonitrile chloroform; acetone H2O 5- 10 (mg/mL) pH 4 Acetate buffer 3 - 5 (mg/mL) pH 9 Borate buffer < 1 (mg/mL) 0.1NHCl 1 - 3 (mg/mL) 0.1 N NaOH decomposes (mg/mL) 10% Ethanol 3 - 5 (mg/mL) 95% Ethanol < 1 (mg/mL) Methanol < 1 (mg/mL) |
Synonyms |
Acetate, Mitoxantrone; CL 232325; CL-232325; CL232325; DHAQ; Hydrochloride, Mitoxantrone; Mitoxantrone; Mitoxantrone Acetate; Mitoxantrone Hydrochloride; Mitozantrone; Mitroxone; Novantron; Novantrone; NSC 279836; NSC 287836; NSC 299195; NSC 301739; NSC 301739D; NSC-279836; NSC-287836; NSC-299195; NSC-301739; NSC-301739D; NSC279836; NSC287836; NSC299195; NSC301739; NSC301739D; Onkotrone; Pralifan; Ralenova |
Origin of Product |
United States |
Retrosynthesis Analysis
AI-Powered Synthesis Planning: Our tool employs the Template_relevance Pistachio, Template_relevance Bkms_metabolic, Template_relevance Pistachio_ringbreaker, Template_relevance Reaxys, Template_relevance Reaxys_biocatalysis model, leveraging a vast database of chemical reactions to predict feasible synthetic routes.
One-Step Synthesis Focus: Specifically designed for one-step synthesis, it provides concise and direct routes for your target compounds, streamlining the synthesis process.
Accurate Predictions: Utilizing the extensive PISTACHIO, BKMS_METABOLIC, PISTACHIO_RINGBREAKER, REAXYS, REAXYS_BIOCATALYSIS database, our tool offers high-accuracy predictions, reflecting the latest in chemical research and data.
Strategy Settings
Precursor scoring | Relevance Heuristic |
---|---|
Min. plausibility | 0.01 |
Model | Template_relevance |
Template Set | Pistachio/Bkms_metabolic/Pistachio_ringbreaker/Reaxys/Reaxys_biocatalysis |
Top-N result to add to graph | 6 |
Feasible Synthetic Routes
Disclaimer and Information on In-Vitro Research Products
Please be aware that all articles and product information presented on BenchChem are intended solely for informational purposes. The products available for purchase on BenchChem are specifically designed for in-vitro studies, which are conducted outside of living organisms. In-vitro studies, derived from the Latin term "in glass," involve experiments performed in controlled laboratory settings using cells or tissues. It is important to note that these products are not categorized as medicines or drugs, and they have not received approval from the FDA for the prevention, treatment, or cure of any medical condition, ailment, or disease. We must emphasize that any form of bodily introduction of these products into humans or animals is strictly prohibited by law. It is essential to adhere to these guidelines to ensure compliance with legal and ethical standards in research and experimentation.