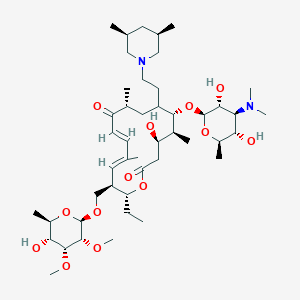
Tilmicosin
Overview
Description
Tilmicosin is a macrolide antibiotic primarily used in veterinary medicine. It is known for its effectiveness in treating bovine respiratory disease and enzootic pneumonia caused by Mannheimia haemolytica in sheep . This compound is distributed under the brand name Micotil and is characterized by its 16-membered lactone ring structure .
Mechanism of Action
Tilmicosin, also known as Micotil, is a semi-synthetic macrolide antibiotic used in veterinary medicine for the treatment of bovine and ovine respiratory diseases .
Target of Action
This compound primarily targets the 50S subunit of the bacterial ribosome . It also inhibits the ArlS histidine kinase activity in Staphylococcus aureus .
Mode of Action
This compound binds to the 50S subunit of the bacterial ribosome, inhibiting protein synthesis . It also inhibits the ArlS histidine kinase activity, which is a part of the unique ArlRS regulatory system in S. aureus .
Biochemical Pathways
This compound affects several biochemical pathways. It induces hepatic and renal oxidative stress, leading to an increase in malondialdehyde levels and a decrease in catalase and reduced glutathione activities . It also affects the expression of several genes in the liver and kidneys .
Pharmacokinetics
It’s known that the compound is used in veterinary medicine, indicating that it has been formulated for effective delivery and absorption in animals .
Result of Action
This compound has potent anti-biofilm activity, disrupting mature biofilms and having a strong bactericidal effect on embedded bacteria . It also modulates the innate immune response, preserving casein production in bovine mammary alveolar cells during S. aureus infection .
Action Environment
The action of this compound can be influenced by environmental factors. For instance, in a BioFlux flow biofilm assay, this compound showed potent anti-biofilm activity and synergized with oxacillin against S. aureus . This suggests that the flow conditions of the environment can influence the efficacy of this compound.
Biochemical Analysis
Biochemical Properties
Tilmicosin interacts with various biomolecules, primarily through its role as a macrolide antibiotic . It inhibits protein synthesis in susceptible bacteria by binding to the 50S subunits in the ribosome, blocking transpeptidation and/or mRNA displacement .
Cellular Effects
This compound has been shown to have significant effects on various types of cells. For instance, it has been found to induce hepatic and renal oxidative stress, increasing malondialdehyde levels while decreasing catalase and reduced glutathione activities . In addition, this compound has been observed to inhibit the expression of certain genes in the S. aureus USA300 strain, demonstrating its influence on gene expression .
Molecular Mechanism
This compound exerts its effects at the molecular level primarily through its inhibition of protein synthesis. It binds to the 50S subunits in the ribosome of susceptible bacteria, blocking transpeptidation and/or mRNA displacement . This disrupts the normal function of the bacteria, leading to their eventual death .
Temporal Effects in Laboratory Settings
In laboratory settings, this compound has been observed to rapidly increase to high levels within 4 hours of administration and remain stable until 48 hours after administration . This suggests that this compound has a relatively long-lasting effect on cellular function.
Dosage Effects in Animal Models
In animal models, the effects of this compound have been observed to vary with different dosages. For instance, in a study conducted on pigs, it was found that a dose of 30, 40, 50, and 60 mg/kg b.w., once daily for 3 days, had significant effects on the population of P. multocida .
Metabolic Pathways
This compound is involved in several metabolic pathways. It is partly desmethylated (yielding T-1), hydroxylated (yielding T-3), or reduced and sulfated (yielding T-4) . Another metabolic pathway in chickens is reductive glycosidic cleavage of the mycinose sugar moiety, yielding metabolites T-9 and T-10 .
Transport and Distribution
This compound is transported and distributed within cells and tissues in a manner that is strongly pH-dependent . It has been found to be mainly distributed to the liver and kidney, with high levels also found in bile, indicating a substantial biliary excretion .
Subcellular Localization
The subcellular localization of this compound has been observed to be primarily in the lysosomes . This localization may play a significant role in its activity and function within the cell.
Preparation Methods
Synthetic Routes and Reaction Conditions: Tilmicosin is synthesized from tylosin tartrate through a series of chemical reactions. The preparation method involves hydrolysis under acidic conditions, followed by alkalization extraction, amination, and phosphorylation with phosphoric pentoxide and a small amount of water. The final product, this compound phosphate, is obtained through full stirring salification, centrifugation separation after solid precipitation, and vacuum drying .
Industrial Production Methods: In industrial settings, this compound is produced using high-performance liquid chromatography (HPLC) with ion-pairing techniques. The process involves liquid-liquid extraction, where this compound remains in a weakly acidic aqueous solution while impurities are removed in a non-polar organic phase. The final partition is performed after making the remaining aqueous phase basic, and this compound is collected in the organic phase .
Chemical Reactions Analysis
Types of Reactions: Tilmicosin undergoes various chemical reactions, including oxidation, reduction, and substitution. It is known for its basic character, which is utilized in its extraction and purification processes .
Common Reagents and Conditions: Common reagents used in the reactions involving this compound include dibutylammonium phosphate, acetonitrile, and phosphoric pentoxide. The conditions often involve acidic or basic environments to facilitate the desired chemical transformations .
Major Products Formed: The major products formed from the reactions involving this compound include its phosphate derivative and other related compounds used in veterinary medicine .
Scientific Research Applications
Tilmicosin has a wide range of scientific research applications. It is used in the development of monoclonal antibodies for immunoassays, which are crucial for detecting residues in milk and animal tissues . Additionally, this compound-loaded polymeric nanoparticles have been developed to improve its bioavailability and practical applications . In microbiology, this compound is used to study its effects on bacterial protein synthesis and its potential as an antibacterial agent .
Comparison with Similar Compounds
Tilmicosin is a semi-synthetic derivative of tylosin, another macrolide antibiotic. Both compounds share a similar antibacterial spectrum and are used as feed additives to promote animal growth . this compound has enhanced activity compared to tylosin, particularly in its coordination with copper(II) complexes . Other similar compounds include erythromycin and azithromycin, which also belong to the macrolide class but differ in their specific structures and applications.
List of Similar Compounds:- Tylosin
- Erythromycin
- Azithromycin
This compound’s unique properties, such as its enhanced activity and specific applications in veterinary medicine, make it a valuable compound in the field of antibiotics.
Properties
CAS No. |
108050-54-0 |
---|---|
Molecular Formula |
C46H80N2O13 |
Molecular Weight |
869.1 g/mol |
IUPAC Name |
(4R,5S,6S,7R,9R,11E,13E,15R,16R)-6-[(3R,4S,5S,6R)-4-(dimethylamino)-3,5-dihydroxy-6-methyloxan-2-yl]oxy-7-[2-[(3R,5S)-3,5-dimethylpiperidin-1-yl]ethyl]-16-ethyl-4-hydroxy-15-[[(2R,3R,4R,5R,6R)-5-hydroxy-3,4-dimethoxy-6-methyloxan-2-yl]oxymethyl]-5,9,13-trimethyl-1-oxacyclohexadeca-11,13-diene-2,10-dione |
InChI |
InChI=1S/C46H80N2O13/c1-13-36-33(24-57-46-44(56-12)43(55-11)40(53)31(8)59-46)19-25(2)14-15-34(49)28(5)20-32(16-17-48-22-26(3)18-27(4)23-48)42(29(6)35(50)21-37(51)60-36)61-45-41(54)38(47(9)10)39(52)30(7)58-45/h14-15,19,26-33,35-36,38-46,50,52-54H,13,16-18,20-24H2,1-12H3/b15-14+,25-19+/t26-,27+,28-,29+,30-,31-,32+,33-,35-,36-,38+,39-,40-,41-,42-,43-,44-,45?,46-/m1/s1 |
InChI Key |
JTSDBFGMPLKDCD-FXBZMSTBSA-N |
Isomeric SMILES |
CC[C@@H]1[C@H](/C=C(/C=C/C(=O)[C@@H](C[C@@H]([C@@H]([C@H]([C@@H](CC(=O)O1)O)C)OC2[C@@H]([C@H]([C@@H]([C@H](O2)C)O)N(C)C)O)CCN3C[C@@H](C[C@@H](C3)C)C)C)\C)CO[C@H]4[C@@H]([C@@H]([C@@H]([C@H](O4)C)O)OC)OC |
SMILES |
CCC1C(C=C(C=CC(=O)C(CC(C(C(C(CC(=O)O1)O)C)OC2C(C(C(C(O2)C)O)N(C)C)O)CCN3CC(CC(C3)C)C)C)C)COC4C(C(C(C(O4)C)O)OC)OC |
Canonical SMILES |
CCC1C(C=C(C=CC(=O)C(CC(C(C(C(CC(=O)O1)O)C)OC2C(C(C(C(O2)C)O)N(C)C)O)CCN3CC(CC(C3)C)C)C)C)COC4C(C(C(C(O4)C)O)OC)OC |
108050-54-0 | |
Pictograms |
Irritant; Health Hazard; Environmental Hazard |
solubility |
In water, 1.5X10-2 mg/L at 25 °C (est) |
Synonyms |
20-deoxo-20-(3,5-dimethylpiperidin-1-yl)desmycosin EL 870 EL-870 MICOTIL 300 tilmicosin |
vapor_pressure |
4.6X10-32 mm Hg at 25 °C (est) |
Origin of Product |
United States |
Retrosynthesis Analysis
AI-Powered Synthesis Planning: Our tool employs the Template_relevance Pistachio, Template_relevance Bkms_metabolic, Template_relevance Pistachio_ringbreaker, Template_relevance Reaxys, Template_relevance Reaxys_biocatalysis model, leveraging a vast database of chemical reactions to predict feasible synthetic routes.
One-Step Synthesis Focus: Specifically designed for one-step synthesis, it provides concise and direct routes for your target compounds, streamlining the synthesis process.
Accurate Predictions: Utilizing the extensive PISTACHIO, BKMS_METABOLIC, PISTACHIO_RINGBREAKER, REAXYS, REAXYS_BIOCATALYSIS database, our tool offers high-accuracy predictions, reflecting the latest in chemical research and data.
Strategy Settings
Precursor scoring | Relevance Heuristic |
---|---|
Min. plausibility | 0.01 |
Model | Template_relevance |
Template Set | Pistachio/Bkms_metabolic/Pistachio_ringbreaker/Reaxys/Reaxys_biocatalysis |
Top-N result to add to graph | 6 |
Feasible Synthetic Routes
Q1: How does tilmicosin exert its antibacterial effect?
A1: this compound binds to the 50S ribosomal subunit of susceptible bacteria, primarily inhibiting protein synthesis. [, , ] This leads to bacterial growth inhibition and eventual death.
Q2: Are there any other effects of this compound beyond its antibacterial action?
A2: Research suggests this compound may have immunomodulatory effects. For example, it has been shown to induce apoptosis (programmed cell death) in bovine neutrophils [], potentially contributing to the resolution of inflammation.
Q3: Does the presence of bacteria influence this compound's ability to induce apoptosis in neutrophils?
A3: Studies indicate that this compound induces apoptosis in bovine neutrophils regardless of the presence or absence of live Pasteurella haemolytica, a common respiratory pathogen. []
Q4: What is the molecular formula and weight of this compound?
A4: this compound has a molecular formula of C46H80NO13.HCl and a molecular weight of 866.5 g/mol. []
Q5: How is this compound absorbed, distributed, metabolized, and excreted in animals?
A5: this compound exhibits rapid absorption following subcutaneous and intramuscular administration in cattle and pigs. [, , ] It is widely distributed in tissues, with a high affinity for the lungs. [, ] Metabolism data is limited, but it is primarily excreted in bile and feces. []
Q6: Does the presence of infection alter the pharmacokinetics of this compound?
A6: Yes, research shows that infection with Actinobacillus pleuropneumoniae in pigs alters this compound's pharmacokinetic parameters, leading to longer drug persistence and greater absorption. []
Q7: What is the in vitro efficacy of this compound against common respiratory pathogens?
A7: this compound demonstrates potent in vitro activity against various bacteria, including Mannheimia haemolytica, Pasteurella multocida, and Mycoplasma spp. [, , ]
Q8: Has the efficacy of this compound been evaluated in clinical settings?
A8: Yes, numerous studies have demonstrated the efficacy of this compound in treating respiratory diseases in cattle and swine under field conditions. [, , ]
Q9: Are there documented cases of resistance to this compound?
A9: Yes, although relatively uncommon, resistance to this compound has been reported in bacteria such as Mannheimia haemolytica and Pasteurella multocida. []
Q10: What are the potential mechanisms of resistance to this compound?
A10: Resistance mechanisms may involve mutations in the 23S rRNA gene, affecting this compound's binding site on the ribosome. []
Q11: Does this compound exhibit cross-resistance with other antimicrobial agents?
A11: As a macrolide antibiotic, this compound may exhibit cross-resistance with other macrolides due to shared mechanisms of action and resistance. []
Q12: What are the potential adverse effects of this compound?
A12: Information regarding adverse effects is detailed in the product literature and should be reviewed for a comprehensive understanding.
Q13: Are there novel drug delivery systems being explored for this compound?
A13: Yes, researchers are investigating the use of nanoparticles as carriers for this compound to enhance its delivery and efficacy. For example, hydrogenated castor oil nanoparticles have shown promise in providing sustained release and improved pharmacokinetic profiles in mice. []
Q14: How are this compound concentrations measured in biological samples?
A14: Various analytical techniques, including high-performance liquid chromatography (HPLC) and microbiological assays, are employed to quantify this compound levels in plasma, tissues, and other biological matrices. [, , ]
Q15: Are there any innovative techniques being used to study this compound distribution in real-time?
A15: Yes, microdialysis, a continuous sampling technique, has been successfully used to investigate this compound pharmacokinetics directly in the joint fluid of chickens, providing valuable insights into drug penetration and distribution to target tissues. []
Q16: What is the potential environmental impact of this compound use?
A16: As with many pharmaceuticals, the environmental fate and potential ecological effects of this compound require further investigation. Responsible disposal practices are crucial to minimize any potential adverse impact on the environment.
Q17: Are there studies on the impact of feed additives on this compound pharmacokinetics?
A17: Yes, research has shown that certain anticoccidial agents, such as amprolium and diclazuril, can significantly alter the pharmacokinetic parameters of this compound in broiler chickens. []
Q18: Is there evidence of this compound’s potential use in other animal species?
A18: While primarily used in cattle and swine, studies have explored the pharmacokinetics and efficacy of this compound in other species like rabbits and donkeys, demonstrating its potential broader application in veterinary medicine. [, ]
Disclaimer and Information on In-Vitro Research Products
Please be aware that all articles and product information presented on BenchChem are intended solely for informational purposes. The products available for purchase on BenchChem are specifically designed for in-vitro studies, which are conducted outside of living organisms. In-vitro studies, derived from the Latin term "in glass," involve experiments performed in controlled laboratory settings using cells or tissues. It is important to note that these products are not categorized as medicines or drugs, and they have not received approval from the FDA for the prevention, treatment, or cure of any medical condition, ailment, or disease. We must emphasize that any form of bodily introduction of these products into humans or animals is strictly prohibited by law. It is essential to adhere to these guidelines to ensure compliance with legal and ethical standards in research and experimentation.