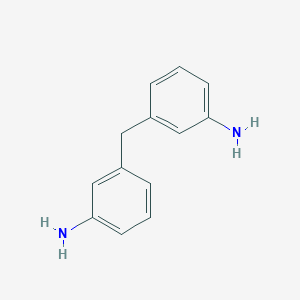
3,3'-Diaminodiphenylmethane
Overview
Description
3,3’-Diaminodiphenylmethane is an organic compound with the chemical formula C₁₃H₁₄N₂. It is a white to pale yellow crystalline solid that is soluble in organic solvents such as chloroform, benzene, and dimethylformamide
Mechanism of Action
Target of Action
It’s known that this compound is used extensively in the preparation of various polymer products .
Mode of Action
The mode of action of 3,3’-Diaminodiphenylmethane involves its interaction with other compounds during the synthesis process. For instance, in the synthesis of 3,3′-dichloro-4,4′-diaminodiphenyl methane (MOCA), 3,3’-Diaminodiphenylmethane interacts with o-chloroaniline and formaldehyde . The exact interaction mechanism is complex and involves several steps .
Biochemical Pathways
It’s known that the compound plays a crucial role in the synthesis of various polymers .
Pharmacokinetics
Given its use in polymer synthesis, it’s likely that these properties would be significantly influenced by the specific conditions of the synthesis process .
Result of Action
The result of 3,3’-Diaminodiphenylmethane’s action is the formation of various polymer products. For instance, it plays a vital role in preparing high-quality polyurethane and epoxy resin .
Preparation Methods
Synthetic Routes and Reaction Conditions
3,3’-Diaminodiphenylmethane can be synthesized through the condensation reaction of aniline with formaldehyde. The reaction typically requires acidic conditions to proceed efficiently. The general reaction scheme involves the formation of a Schiff base intermediate, which is subsequently reduced to yield the final product .
Industrial Production Methods
In industrial settings, the production of 3,3’-Diaminodiphenylmethane often involves the use of solid acid catalysts to enhance the reaction rate and selectivity. The reaction is carried out at elevated temperatures, typically between 120°C and 200°C, and under controlled pressure conditions . The use of solid acid catalysts also allows for the recycling of unreacted starting materials, making the process more environmentally friendly.
Chemical Reactions Analysis
Types of Reactions
3,3’-Diaminodiphenylmethane undergoes various chemical reactions, including:
Oxidation: This compound can be oxidized to form quinone derivatives.
Reduction: It can be reduced to form corresponding amines.
Substitution: It can undergo electrophilic substitution reactions, particularly at the aromatic rings.
Common Reagents and Conditions
Oxidation: Common oxidizing agents include potassium permanganate and chromium trioxide.
Reduction: Reducing agents such as lithium aluminum hydride or hydrogen gas in the presence of a catalyst are commonly used.
Substitution: Electrophilic substitution reactions often require the presence of a Lewis acid catalyst.
Major Products
Oxidation: Quinone derivatives.
Reduction: Corresponding amines.
Substitution: Various substituted aromatic compounds depending on the electrophile used.
Scientific Research Applications
3,3’-Diaminodiphenylmethane has a wide range of applications in scientific research and industry:
Biology: It is used in the preparation of bioactive molecules and as a cross-linking agent in the study of protein structures.
Industry: It is used as a curing agent for epoxy resins and as a hardener for polyurethane systems.
Comparison with Similar Compounds
Similar Compounds
4,4’-Diaminodiphenylmethane: Similar in structure but with amino groups at the para positions.
3,3’-Dichloro-4,4’-Diaminodiphenylmethane: Contains chlorine substituents, which alter its reactivity and applications.
3,3’-Dimethyl-4,4’-Diaminodiphenylmethane: Contains methyl groups, which affect its physical and chemical properties.
Uniqueness
3,3’-Diaminodiphenylmethane is unique due to its specific substitution pattern, which influences its reactivity and makes it suitable for particular applications in polymer and resin production. Its ability to form stable intermediates and final products under various reaction conditions also sets it apart from other similar compounds .
Properties
IUPAC Name |
3-[(3-aminophenyl)methyl]aniline | |
---|---|---|
Source | PubChem | |
URL | https://pubchem.ncbi.nlm.nih.gov | |
Description | Data deposited in or computed by PubChem | |
InChI |
InChI=1S/C13H14N2/c14-12-5-1-3-10(8-12)7-11-4-2-6-13(15)9-11/h1-6,8-9H,7,14-15H2 | |
Source | PubChem | |
URL | https://pubchem.ncbi.nlm.nih.gov | |
Description | Data deposited in or computed by PubChem | |
InChI Key |
CKOFBUUFHALZGK-UHFFFAOYSA-N | |
Source | PubChem | |
URL | https://pubchem.ncbi.nlm.nih.gov | |
Description | Data deposited in or computed by PubChem | |
Canonical SMILES |
C1=CC(=CC(=C1)N)CC2=CC(=CC=C2)N | |
Source | PubChem | |
URL | https://pubchem.ncbi.nlm.nih.gov | |
Description | Data deposited in or computed by PubChem | |
Molecular Formula |
C13H14N2 | |
Source | PubChem | |
URL | https://pubchem.ncbi.nlm.nih.gov | |
Description | Data deposited in or computed by PubChem | |
DSSTOX Substance ID |
DTXSID30173110 | |
Record name | Benzenamine, 3,3'-methylenebis- | |
Source | EPA DSSTox | |
URL | https://comptox.epa.gov/dashboard/DTXSID30173110 | |
Description | DSSTox provides a high quality public chemistry resource for supporting improved predictive toxicology. | |
Molecular Weight |
198.26 g/mol | |
Source | PubChem | |
URL | https://pubchem.ncbi.nlm.nih.gov | |
Description | Data deposited in or computed by PubChem | |
CAS No. |
19471-12-6 | |
Record name | Benzenamine, 3,3'-methylenebis- | |
Source | ChemIDplus | |
URL | https://pubchem.ncbi.nlm.nih.gov/substance/?source=chemidplus&sourceid=0019471126 | |
Description | ChemIDplus is a free, web search system that provides access to the structure and nomenclature authority files used for the identification of chemical substances cited in National Library of Medicine (NLM) databases, including the TOXNET system. | |
Record name | Benzenamine, 3,3'-methylenebis- | |
Source | EPA DSSTox | |
URL | https://comptox.epa.gov/dashboard/DTXSID30173110 | |
Description | DSSTox provides a high quality public chemistry resource for supporting improved predictive toxicology. | |
Record name | 3,3'-Methylenedianiline | |
Source | European Chemicals Agency (ECHA) | |
URL | https://echa.europa.eu/information-on-chemicals | |
Description | The European Chemicals Agency (ECHA) is an agency of the European Union which is the driving force among regulatory authorities in implementing the EU's groundbreaking chemicals legislation for the benefit of human health and the environment as well as for innovation and competitiveness. | |
Explanation | Use of the information, documents and data from the ECHA website is subject to the terms and conditions of this Legal Notice, and subject to other binding limitations provided for under applicable law, the information, documents and data made available on the ECHA website may be reproduced, distributed and/or used, totally or in part, for non-commercial purposes provided that ECHA is acknowledged as the source: "Source: European Chemicals Agency, http://echa.europa.eu/". Such acknowledgement must be included in each copy of the material. ECHA permits and encourages organisations and individuals to create links to the ECHA website under the following cumulative conditions: Links can only be made to webpages that provide a link to the Legal Notice page. | |
Synthesis routes and methods I
Procedure details
Synthesis routes and methods II
Procedure details
Retrosynthesis Analysis
AI-Powered Synthesis Planning: Our tool employs the Template_relevance Pistachio, Template_relevance Bkms_metabolic, Template_relevance Pistachio_ringbreaker, Template_relevance Reaxys, Template_relevance Reaxys_biocatalysis model, leveraging a vast database of chemical reactions to predict feasible synthetic routes.
One-Step Synthesis Focus: Specifically designed for one-step synthesis, it provides concise and direct routes for your target compounds, streamlining the synthesis process.
Accurate Predictions: Utilizing the extensive PISTACHIO, BKMS_METABOLIC, PISTACHIO_RINGBREAKER, REAXYS, REAXYS_BIOCATALYSIS database, our tool offers high-accuracy predictions, reflecting the latest in chemical research and data.
Strategy Settings
Precursor scoring | Relevance Heuristic |
---|---|
Min. plausibility | 0.01 |
Model | Template_relevance |
Template Set | Pistachio/Bkms_metabolic/Pistachio_ringbreaker/Reaxys/Reaxys_biocatalysis |
Top-N result to add to graph | 6 |
Feasible Synthetic Routes
Q1: What is the primary application of 3,3'-Diaminodiphenylmethane in the context of the provided research?
A1: The provided research highlights this compound as a crucial component in synthesizing polyamide acid and polyimide. [] This compound serves as an aromatic diamine, reacting with tetracarboxylic dianhydrides to form these polymers. These polymers find applications as adhesives or bonding agents. [] Additionally, this compound is identified as a significant degradation product of rigid polyurethane (PU) and polyisocyanurate (PIR) foams, particularly those derived from renewable materials like tall oil fatty acid-based polyols. []
Q2: How does the structure of this compound influence its interaction with poly(ε-caprolactone) (PCL)?
A2: Research indicates that this compound forms strong intermolecular hydrogen bonds with poly(ε-caprolactone) (PCL). [] This interaction arises from the presence of two amino groups in this compound, which can form hydrogen bonds with the carbonyl groups present in PCL. This interaction significantly impacts the thermal and mechanical properties of PCL blends. For instance, increasing the concentration of this compound leads to a higher glass transition temperature but a lower melting point and reduced elongation-at-break in the blend. []
Q3: What are the safety concerns associated with this compound, especially in the context of its presence in the pyrolysis products of specific foams?
A3: Analysis of pyrolysis products from rigid PU and PIR foams identified this compound as a major component. [] This compound, alongside other degradation products like 4,4′-methylenedianiline, exhibits health hazards, including skin and eye irritation, respiratory irritation, and acute toxicity. [] Notably, 4,4′-methylenedianiline, the most abundant pyrolysis product, is classified as a suspected carcinogen and mutagen. [] These findings underscore the importance of understanding the potential health risks associated with the thermal degradation of these foams and emphasize the need for appropriate safety measures during handling and disposal.
Disclaimer and Information on In-Vitro Research Products
Please be aware that all articles and product information presented on BenchChem are intended solely for informational purposes. The products available for purchase on BenchChem are specifically designed for in-vitro studies, which are conducted outside of living organisms. In-vitro studies, derived from the Latin term "in glass," involve experiments performed in controlled laboratory settings using cells or tissues. It is important to note that these products are not categorized as medicines or drugs, and they have not received approval from the FDA for the prevention, treatment, or cure of any medical condition, ailment, or disease. We must emphasize that any form of bodily introduction of these products into humans or animals is strictly prohibited by law. It is essential to adhere to these guidelines to ensure compliance with legal and ethical standards in research and experimentation.