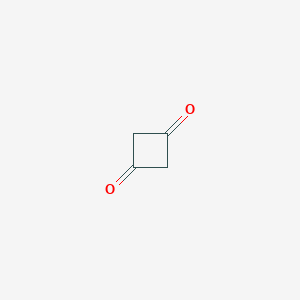
Cyclobutane-1,3-dione
Overview
Description
Cyclobutane-1,3-dione (C₄H₄O₂; molecular weight: 84.07 g/mol) is a strained cyclic diketone characterized by a four-membered cyclobutane ring with two ketone groups at the 1 and 3 positions. Key physical properties include a boiling point of 226.9°C, density of 1.314 g/cm³, and vapor pressure of 0.08 mmHg at 25°C . Its synthesis involves methods such as U(III)-mediated activation of carbon suboxide (C₃O₂) and thermolysis of intermediates like 3-ethoxy-2-cyclobutenone . This compound serves as a precursor in organic synthesis, enabling access to alkaloids (e.g., (–)-huperzine A) and novel secondary metabolites via engineered polyketide synthases .
Preparation Methods
Synthetic Routes and Reaction Conditions: Cyclobutane-1,3-dione can be synthesized through various methods. One common approach involves the cyclization of 2-methyl-3-phenylpropanoic acid. The process begins with the conversion of the acid to its corresponding acid chloride using thionyl chloride (SOCl₂) and dimethylformamide (DMF). The acid chloride is then reacted with ethoxyacetylene in the presence of triethylamine to form an intermediate, which is subsequently hydrolyzed to yield this compound .
Industrial Production Methods: While specific industrial production methods for this compound are not extensively documented, the synthesis typically involves similar organic reactions scaled up for industrial use. The choice of reagents and conditions would be optimized for yield, cost, and safety.
Chemical Reactions Analysis
Types of Reactions: Cyclobutane-1,3-dione undergoes various chemical reactions, including:
Oxidation: It can be oxidized to form more complex diketones or carboxylic acids.
Reduction: Reduction reactions can convert the diketone to cyclobutane-1,3-diol.
Substitution: The compound can participate in nucleophilic substitution reactions, where one of the carbonyl groups is replaced by other functional groups.
Common Reagents and Conditions:
Oxidation: Common oxidizing agents include potassium permanganate (KMnO₄) and chromium trioxide (CrO₃).
Reduction: Reducing agents such as lithium aluminum hydride (LiAlH₄) or sodium borohydride (NaBH₄) are typically used.
Substitution: Nucleophiles like amines or alcohols can be used under acidic or basic conditions.
Major Products:
Oxidation: Products include carboxylic acids or more complex diketones.
Reduction: The major product is cyclobutane-1,3-diol.
Substitution: Products vary depending on the nucleophile used, resulting in substituted cyclobutane derivatives.
Scientific Research Applications
Organic Synthesis
Building Block in Organic Chemistry
Cyclobutane-1,3-dione serves as a critical intermediate in organic synthesis. Its structural characteristics allow it to participate in various chemical reactions, including:
- Condensation Reactions : It can undergo condensation with amines to form chiral compounds, as demonstrated in studies utilizing chiral phosphoric acid catalysts .
- Reduction and Oxidation : The compound can be reduced to cyclobutane-1,3-diol or oxidized to yield more complex diketones or carboxylic acids.
These reactions facilitate the construction of more complex cyclic structures, which are essential in synthesizing pharmaceuticals and agrochemicals.
Medicinal Chemistry
Potential Antimicrobial and Anticancer Properties
Research has indicated that derivatives of this compound may exhibit significant biological activity. Studies have highlighted its potential as a lead compound against various pathogens:
- Antitubercular Activity : Recent investigations into cyclobutene derivatives related to this compound have shown promising results as inhibitors against Mycobacterium tuberculosis ATP synthase, suggesting potential for drug development against tuberculosis .
- Anticancer Research : The structural properties of this compound allow for modifications that may enhance its efficacy against cancer cells. Ongoing research is exploring its derivatives for their anticancer activities.
Materials Science
Applications in Polymer Chemistry
The unique reactivity of this compound makes it suitable for applications in materials science:
- Synthesis of Polymers : Its ability to undergo polymerization reactions allows this compound to be incorporated into polymer matrices, potentially leading to materials with enhanced properties.
- Development of Functional Materials : The compound's structural features may enable the design of new functional materials with specific electronic or optical properties.
Case Study 1: Enantioselective Condensation
A study published in Organic Letters demonstrated the enantioselective condensation of cyclobutane-1,3-diones with primary amines using chiral phosphoric acid catalysts . This method showcases the compound's utility in synthesizing optically active compounds that are vital for pharmaceutical applications.
Case Study 2: Antitubercular Derivatives
Research on cyclobutene derivatives has revealed their potential as effective inhibitors against Mycobacterium tuberculosis. Molecular docking studies indicated favorable binding affinities towards ATP synthase, paving the way for further drug development efforts against tuberculosis .
Mechanism of Action
Cyclobutane-1,3-dione can be compared with other cyclic diketones such as cyclopentanedione and cyclohexanedione:
Cyclopentanedione: This compound has a five-membered ring and exhibits different reactivity due to reduced ring strain compared to this compound.
Cyclohexanedione: With a six-membered ring, cyclohexanedione is even less strained and shows different chemical behavior.
Uniqueness: this compound is unique due to its high ring strain and the presence of two carbonyl groups in a four-membered ring, making it more reactive and versatile in chemical synthesis compared to its larger ring counterparts .
Comparison with Similar Compounds
Comparison with Structurally Similar Compounds
Cyclohexane-1,3-dione
- Structure and Stability : The six-membered cyclohexane ring reduces angle strain compared to cyclobutane-1,3-dione, resulting in greater thermodynamic stability.
- Applications: Cyclohexane-1,3-dione derivatives exhibit notable antibacterial activity. For example, compounds 5a–5h (Table 3 in ) show efficacy against strains like Staphylococcus aureus and Escherichia coli.
- Reactivity : Participates in multi-component reactions (e.g., with benzaldehyde and dimedone) to form hexahydroxanthenedione derivatives .
Cyclopentane-1,3-dione
- Role as Isostere : Functions as a bioisostere for carboxylic acids due to its similar pKa (~5.5) and hydrogen-bonding capacity, enabling use in drug design .
- Crystallography : X-ray studies reveal planar geometries, with intramolecular hydrogen bonding stabilizing the dione form .
Substituted Cyclobutane Diones
- 2-Chlorothis compound (C₄H₃ClO₂; MW: 118.52 g/mol): The electron-withdrawing chlorine substituent enhances electrophilicity, facilitating nucleophilic attacks. Used in halogenation studies .
Comparative Data Table
Key Research Findings
- Thermodynamic Stability : this compound and diketene exhibit similar heats of combustion (Table I in ), suggesting comparable ground-state stability. However, thermolysis of this compound yields 53% ketene at 400°C, while diketene produces 83% under identical conditions, indicating distinct decomposition pathways .
- Ring Strain Effects : The cyclobutane ring’s high angle strain (~90° bond angles) enhances reactivity in [2+2] cycloadditions compared to larger rings .
- Biological Relevance : Cyclohexane-1,3-dione derivatives demonstrate broader pharmaceutical applicability (e.g., antimicrobial activity) than this compound, which is primarily used in synthetic chemistry .
Biological Activity
Cyclobutane-1,3-dione, a cyclic dione, has garnered attention in the field of medicinal chemistry due to its diverse biological activities. This article synthesizes findings from various studies to provide a comprehensive overview of the biological activity associated with this compound, including its antimicrobial properties and potential applications in therapeutic contexts.
Chemical Structure and Properties
This compound features a four-membered ring structure with two carbonyl groups positioned at the 1 and 3 positions. Its unique structure contributes to its reactivity and biological activity. The compound can undergo various chemical reactions, leading to the formation of derivatives with enhanced or altered biological properties.
Antimicrobial Activity
Recent studies have highlighted the antimicrobial potential of this compound and its derivatives. For instance, a study published in the Egyptian Journal of Chemistry evaluated the antimicrobial activity of several synthesized compounds derived from this compound. The results indicated that specific derivatives exhibited significant antibacterial and antifungal activities against various pathogens.
Table 1: Antimicrobial Activity of this compound Derivatives
Compound No. | Microorganism | Inhibition Zone Diameter (mm) |
---|---|---|
1 | Staphylococcus aureus | 22 |
2 | Escherichia coli | 18 |
3 | Bacillus cereus | 15 |
4 | Pseudomonas aeruginosa | 17 |
5 | Candida albicans | 20 |
The above table summarizes the inhibition zones observed for different derivatives against selected microorganisms. Notably, compound 1 showed the highest activity against Staphylococcus aureus, indicating its potential as an antimicrobial agent .
Cytotoxicity Studies
In addition to antimicrobial properties, this compound has been investigated for its cytotoxic effects on tumor cell lines. A study assessed the cytotoxicity of various derivatives against human tumor cell lines, revealing that some compounds exhibited selective toxicity towards cancer cells while sparing normal cells.
Table 2: Cytotoxicity of this compound Derivatives
Compound No. | Cell Line | IC50 (µM) |
---|---|---|
1 | HeLa | 12 |
2 | MCF-7 | 15 |
3 | A549 | 10 |
4 | Normal Human Cells | >50 |
The data indicates that compound 3 had the lowest IC50 value against the A549 cell line, suggesting a promising lead for further development in cancer therapy .
The mechanism underlying the biological activity of this compound is not fully understood; however, it is hypothesized that its ability to form reactive intermediates plays a crucial role. The carbonyl groups can participate in nucleophilic attacks, leading to interactions with biomolecules such as proteins and nucleic acids.
Case Studies and Applications
Several case studies have explored the applications of this compound in drug design. For example, derivatives have been synthesized for targeted therapies in cancer treatment and as potential antifungal agents. The versatility of this compound allows for modifications that can enhance selectivity and potency against specific targets.
Notable Case Study: Antifungal Activity
A particular derivative of this compound was tested against Candida albicans, demonstrating an inhibition rate comparable to established antifungal drugs. This study underscores the potential for developing new antifungal therapies based on this compound .
Q & A
Basic Research Questions
Q. What are the primary synthetic routes for cyclobutane-1,3-dione, and how do experimental conditions influence yield and purity?
this compound is commonly synthesized via the hydrolysis of 3-ethoxy-2-cyclobutenone, followed by molecular distillation for purification, achieving yields of 50–70% . Alternative methods include uranium(III)-mediated reductive coupling of carbon suboxide (C₃O₂), which forms a this compound ring via trapping of a ketene intermediate . Key factors affecting yield include reaction temperature (e.g., 380°C for thermolysis of diketenes) and carrier gas use (e.g., N₂) to minimize surface decomposition . Purity is validated via NMR, HRMS, and UV spectroscopy .
Q. How is the stereochemistry and conformation of this compound derivatives analyzed experimentally?
Stereochemical analysis relies on 1D NOE (Nuclear Overhauser Effect) experiments to confirm spatial arrangements of substituents. For example, trans-3-(((3-methyloxetan-3-yl)methyl)amino)-3-propylcyclobutan-1-ol was characterized using NOE to validate cis/trans configurations . X-ray crystallography and temperature-dependent NMR can further resolve ring strain effects on conformational flexibility .
Q. What spectroscopic and computational methods are used to characterize this compound’s electronic structure?
Infrared (IR) spectroscopy identifies carbonyl stretching frequencies (~1,750 cm⁻¹), while UV spectroscopy monitors π→π* transitions in ethanol solutions . Computational studies (e.g., semi-empirical molecular orbital methods) model the compound’s reactivity, including its dimerization pathways and activation energies (~50 kcal/mol for thermolysis) .
Advanced Research Questions
Q. How do researchers resolve contradictions in thermolysis data between this compound and its derivatives?
Discrepancies in thermolysis mechanisms (e.g., concerted vs. stepwise pathways) are addressed via isotopic labeling and competitive kinetic experiments. For example, deuterated ketene derivatives were used to rule out scrambling during this compound decomposition, confirming a concerted mechanism with an activation energy of ~31 kcal/mol for ketene dimerization . Parallel studies comparing diketene and this compound thermolysis under controlled carrier gas (N₂) and pressure (140 mmHg) conditions help isolate homogeneous vs. surface-mediated reactions .
Q. What strategies optimize this compound’s stability in fragment-based drug discovery applications?
The compound’s rigid, strained ring system is leveraged to design conformationally restricted pharmacophores. For instance, 3-chloro-N-((cis)-3-hydroxy-1-propylcyclobutyl)thiophene-2-carboxamide was synthesized via fragment coupling, with stability enhanced by steric shielding of reactive carbonyl groups . Accelerated stability testing (e.g., thermal stress at 40–80°C) and crystallographic packing analysis guide the selection of substituents that mitigate ring-opening reactions .
Q. How are computational models employed to predict this compound’s reactivity in novel cycloaddition reactions?
Density functional theory (DFT) calculations predict regioselectivity in [2+2] cycloadditions, such as the head-to-tail dimerization of ketenes to form this compound derivatives. These models incorporate orbital symmetry (Woodward-Hoffmann rules) and strain energy (~27 kcal/mol for the cyclobutane ring) to simulate transition states and reaction barriers . Experimental validation uses kinetic isotope effects (KIEs) and Arrhenius plots derived from flow reactor data .
Q. What thermodynamic data are critical for assessing this compound’s feasibility in synthetic applications?
Key parameters include:
- Heat of combustion : −450.7 ± 0.5 kcal/mol (measured via oxygen bomb calorimetry) .
- Vapor pressure : ~20 × 10⁵ Pa at room temperature, critical for gas-phase reactions .
- Activation energy : 50 kcal/mol for thermolysis, indicating high thermal stability . These data inform process design, such as distillation temperatures and solvent-free reaction conditions .
Q. Methodological Considerations
Q. How should researchers design experiments to minimize side reactions during this compound synthesis?
- Use inert atmospheres (e.g., N₂/Ar) to prevent oxidation of reactive intermediates .
- Employ low-temperature molecular distillation to avoid decomposition of thermally labile precursors .
- Optimize stoichiometry in reductive coupling reactions (e.g., C₃O₂:U(III) ratio) to suppress byproducts like CO .
Q. What analytical workflows are recommended for detecting trace impurities in this compound samples?
Properties
IUPAC Name |
cyclobutane-1,3-dione | |
---|---|---|
Source | PubChem | |
URL | https://pubchem.ncbi.nlm.nih.gov | |
Description | Data deposited in or computed by PubChem | |
InChI |
InChI=1S/C4H4O2/c5-3-1-4(6)2-3/h1-2H2 | |
Source | PubChem | |
URL | https://pubchem.ncbi.nlm.nih.gov | |
Description | Data deposited in or computed by PubChem | |
InChI Key |
PZQGSZRQKQZCOJ-UHFFFAOYSA-N | |
Source | PubChem | |
URL | https://pubchem.ncbi.nlm.nih.gov | |
Description | Data deposited in or computed by PubChem | |
Canonical SMILES |
C1C(=O)CC1=O | |
Source | PubChem | |
URL | https://pubchem.ncbi.nlm.nih.gov | |
Description | Data deposited in or computed by PubChem | |
Molecular Formula |
C4H4O2 | |
Source | PubChem | |
URL | https://pubchem.ncbi.nlm.nih.gov | |
Description | Data deposited in or computed by PubChem | |
DSSTOX Substance ID |
DTXSID80165803 | |
Record name | Cyclobutane-1,3-dione | |
Source | EPA DSSTox | |
URL | https://comptox.epa.gov/dashboard/DTXSID80165803 | |
Description | DSSTox provides a high quality public chemistry resource for supporting improved predictive toxicology. | |
Molecular Weight |
84.07 g/mol | |
Source | PubChem | |
URL | https://pubchem.ncbi.nlm.nih.gov | |
Description | Data deposited in or computed by PubChem | |
CAS No. |
15506-53-3 | |
Record name | Cyclobutane-1,3-dione | |
Source | ChemIDplus | |
URL | https://pubchem.ncbi.nlm.nih.gov/substance/?source=chemidplus&sourceid=0015506533 | |
Description | ChemIDplus is a free, web search system that provides access to the structure and nomenclature authority files used for the identification of chemical substances cited in National Library of Medicine (NLM) databases, including the TOXNET system. | |
Record name | Cyclobutane-1,3-dione | |
Source | EPA DSSTox | |
URL | https://comptox.epa.gov/dashboard/DTXSID80165803 | |
Description | DSSTox provides a high quality public chemistry resource for supporting improved predictive toxicology. | |
Retrosynthesis Analysis
AI-Powered Synthesis Planning: Our tool employs the Template_relevance Pistachio, Template_relevance Bkms_metabolic, Template_relevance Pistachio_ringbreaker, Template_relevance Reaxys, Template_relevance Reaxys_biocatalysis model, leveraging a vast database of chemical reactions to predict feasible synthetic routes.
One-Step Synthesis Focus: Specifically designed for one-step synthesis, it provides concise and direct routes for your target compounds, streamlining the synthesis process.
Accurate Predictions: Utilizing the extensive PISTACHIO, BKMS_METABOLIC, PISTACHIO_RINGBREAKER, REAXYS, REAXYS_BIOCATALYSIS database, our tool offers high-accuracy predictions, reflecting the latest in chemical research and data.
Strategy Settings
Precursor scoring | Relevance Heuristic |
---|---|
Min. plausibility | 0.01 |
Model | Template_relevance |
Template Set | Pistachio/Bkms_metabolic/Pistachio_ringbreaker/Reaxys/Reaxys_biocatalysis |
Top-N result to add to graph | 6 |
Feasible Synthetic Routes
Disclaimer and Information on In-Vitro Research Products
Please be aware that all articles and product information presented on BenchChem are intended solely for informational purposes. The products available for purchase on BenchChem are specifically designed for in-vitro studies, which are conducted outside of living organisms. In-vitro studies, derived from the Latin term "in glass," involve experiments performed in controlled laboratory settings using cells or tissues. It is important to note that these products are not categorized as medicines or drugs, and they have not received approval from the FDA for the prevention, treatment, or cure of any medical condition, ailment, or disease. We must emphasize that any form of bodily introduction of these products into humans or animals is strictly prohibited by law. It is essential to adhere to these guidelines to ensure compliance with legal and ethical standards in research and experimentation.