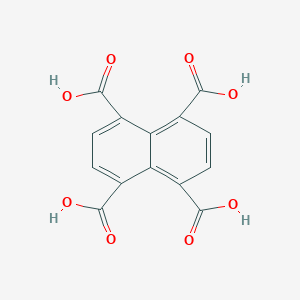
1,4,5,8-Naphthalenetetracarboxylic acid
Overview
Description
1,4,5,8-Naphthalenetetracarboxylic acid is an organic compound with the molecular formula C14H8O8. It is a polycarboxylic acid derived from naphthalene, featuring four carboxyl groups attached to the naphthalene ring system. This compound is known for its applications in organic electronics, dyes, and as a precursor for various chemical syntheses.
Mechanism of Action
Target of Action
1,4,5,8-Naphthalenetetracarboxylic acid (NTCDA) is a highly conjugated, carbonyl-containing molecule . It is primarily used as an electron-transporting material in organic electronics . The compound’s primary targets are the electronic systems of these devices, where it can accept electrons due to its extended conjugated ring system and electron-withdrawing groups .
Mode of Action
The mode of action of NTCDA involves the transmission of electronic effects through its conjugated framework . In basic solution, the hydrolysis of NTCDA yields, sequentially, 1,4,5,8-naphthalene diacid monoanhydride (II), and 1,4,5,8-naphthalene tetracarboxylic acid (I). The second-order rate constant for alkaline hydrolysis is 200-fold higher for the first ring opening .
Pharmacokinetics
It is known that the compound is insoluble in ethanol, chloroform, and benzene, and slightly soluble in acetone aqueous solution .
Result of Action
The result of NTCDA’s action is the improvement of the electric conductivity in co-deposited films of NTCDA and several metals, including indium, magnesium, and aluminum . This can effectively reduce the leakage current and improve the breakdown strength and energy storage properties of the composite at high temperature .
Action Environment
The action of NTCDA is influenced by environmental factors such as pH and temperature . The kinetics of hydrolysis of NTCDA in aqueous solution were determined from 5 M acid to pH 10 . The rate constant for water-catalyzed hydrolysis of NTCDA is 25-fold higher than that for II . In concentrated acid, the rates for reaching equilibrium (I, II, and III) increase, and III is the major product .
Biochemical Analysis
Biochemical Properties
It is known that this compound can interact with various biomolecules in a biochemical context .
Molecular Mechanism
It is known that this compound can interact with various biomolecules at the molecular level .
Dosage Effects in Animal Models
The effects of different dosages of 1,4,5,8-Naphthalenetetracarboxylic acid in animal models have not been extensively studied. Therefore, information about threshold effects, toxic effects at high doses, and other dosage-related effects is currently unavailable .
Preparation Methods
1,4,5,8-Naphthalenetetracarboxylic acid can be synthesized from pyrene through a series of chlorination and oxidation reactions . The process involves the following steps:
Chlorination: Pyrene is chlorinated to form tetrachloropyrene.
Oxidation: The tetrachloropyrene is then oxidized to form this compound.
Industrial production methods typically involve similar steps but are optimized for large-scale synthesis, ensuring high yield and purity of the final product.
Chemical Reactions Analysis
1,4,5,8-Naphthalenetetracarboxylic acid undergoes various chemical reactions, including:
Hydrolysis: In basic solutions, it hydrolyzes to form 1,4,5,8-naphthalene diacid monoanhydride and subsequently this compound.
Oxidation and Reduction: It can be oxidized or reduced under specific conditions to form different derivatives.
Substitution Reactions: The carboxyl groups can participate in substitution reactions, forming esters, amides, and other derivatives.
Common reagents used in these reactions include strong acids, bases, and oxidizing agents. The major products formed depend on the reaction conditions and the reagents used.
Scientific Research Applications
1,4,5,8-Naphthalenetetracarboxylic acid has a wide range of applications in scientific research:
Organic Electronics: It is used as an electron transporting material in organic electronics, including organic photovoltaics and thin-film transistors.
Coordination Polymers: It serves as a ligand in the synthesis of coordination polymers and metal-organic frameworks, which have applications in gas storage, catalysis, and supercapacitors.
Fluorescent Markers: It is used in the preparation of fluorescent markers for biological research.
Battery Materials: It is employed as an anode material in lithium-ion batteries, enhancing their performance and stability.
Comparison with Similar Compounds
1,4,5,8-Naphthalenetetracarboxylic acid is unique due to its four carboxyl groups, which provide multiple sites for chemical reactions and coordination. Similar compounds include:
1,4,5,8-Naphthalenetetracarboxylic dianhydride: This compound is a precursor to this compound and is used in similar applications.
3,4,9,10-Perylenetetracarboxylic dianhydride: Another polycarboxylic acid used in organic electronics and dye synthesis.
Pyromellitic dianhydride: A related compound with four carboxyl groups, used in the production of polyimides and other high-performance polymers.
These compounds share similar chemical properties but differ in their structural frameworks and specific applications.
Properties
IUPAC Name |
naphthalene-1,4,5,8-tetracarboxylic acid | |
---|---|---|
Source | PubChem | |
URL | https://pubchem.ncbi.nlm.nih.gov | |
Description | Data deposited in or computed by PubChem | |
InChI |
InChI=1S/C14H8O8/c15-11(16)5-1-2-6(12(17)18)10-8(14(21)22)4-3-7(9(5)10)13(19)20/h1-4H,(H,15,16)(H,17,18)(H,19,20)(H,21,22) | |
Source | PubChem | |
URL | https://pubchem.ncbi.nlm.nih.gov | |
Description | Data deposited in or computed by PubChem | |
InChI Key |
OLAPPGSPBNVTRF-UHFFFAOYSA-N | |
Source | PubChem | |
URL | https://pubchem.ncbi.nlm.nih.gov | |
Description | Data deposited in or computed by PubChem | |
Canonical SMILES |
C1=CC(=C2C(=CC=C(C2=C1C(=O)O)C(=O)O)C(=O)O)C(=O)O | |
Source | PubChem | |
URL | https://pubchem.ncbi.nlm.nih.gov | |
Description | Data deposited in or computed by PubChem | |
Molecular Formula |
C14H8O8 | |
Source | PubChem | |
URL | https://pubchem.ncbi.nlm.nih.gov | |
Description | Data deposited in or computed by PubChem | |
DSSTOX Substance ID |
DTXSID4059592 | |
Record name | 1,4,5,8-Naphthalenetetracarboxylic acid | |
Source | EPA DSSTox | |
URL | https://comptox.epa.gov/dashboard/DTXSID4059592 | |
Description | DSSTox provides a high quality public chemistry resource for supporting improved predictive toxicology. | |
Molecular Weight |
304.21 g/mol | |
Source | PubChem | |
URL | https://pubchem.ncbi.nlm.nih.gov | |
Description | Data deposited in or computed by PubChem | |
CAS No. |
128-97-2 | |
Record name | 1,4,5,8-Naphthalenetetracarboxylic acid | |
Source | CAS Common Chemistry | |
URL | https://commonchemistry.cas.org/detail?cas_rn=128-97-2 | |
Description | CAS Common Chemistry is an open community resource for accessing chemical information. Nearly 500,000 chemical substances from CAS REGISTRY cover areas of community interest, including common and frequently regulated chemicals, and those relevant to high school and undergraduate chemistry classes. This chemical information, curated by our expert scientists, is provided in alignment with our mission as a division of the American Chemical Society. | |
Explanation | The data from CAS Common Chemistry is provided under a CC-BY-NC 4.0 license, unless otherwise stated. | |
Record name | 1,4,5,8-Naphthalenetetracarboxylic acid | |
Source | ChemIDplus | |
URL | https://pubchem.ncbi.nlm.nih.gov/substance/?source=chemidplus&sourceid=0000128972 | |
Description | ChemIDplus is a free, web search system that provides access to the structure and nomenclature authority files used for the identification of chemical substances cited in National Library of Medicine (NLM) databases, including the TOXNET system. | |
Record name | Tetra acid | |
Source | DTP/NCI | |
URL | https://dtp.cancer.gov/dtpstandard/servlet/dwindex?searchtype=NSC&outputformat=html&searchlist=66207 | |
Description | The NCI Development Therapeutics Program (DTP) provides services and resources to the academic and private-sector research communities worldwide to facilitate the discovery and development of new cancer therapeutic agents. | |
Explanation | Unless otherwise indicated, all text within NCI products is free of copyright and may be reused without our permission. Credit the National Cancer Institute as the source. | |
Record name | 1,4,5,8-Naphthalenetetracarboxylic acid | |
Source | EPA Chemicals under the TSCA | |
URL | https://www.epa.gov/chemicals-under-tsca | |
Description | EPA Chemicals under the Toxic Substances Control Act (TSCA) collection contains information on chemicals and their regulations under TSCA, including non-confidential content from the TSCA Chemical Substance Inventory and Chemical Data Reporting. | |
Record name | 1,4,5,8-Naphthalenetetracarboxylic acid | |
Source | EPA DSSTox | |
URL | https://comptox.epa.gov/dashboard/DTXSID4059592 | |
Description | DSSTox provides a high quality public chemistry resource for supporting improved predictive toxicology. | |
Record name | Naphthalene-1,4,5,8-tetracarboxylic acid | |
Source | European Chemicals Agency (ECHA) | |
URL | https://echa.europa.eu/substance-information/-/substanceinfo/100.004.478 | |
Description | The European Chemicals Agency (ECHA) is an agency of the European Union which is the driving force among regulatory authorities in implementing the EU's groundbreaking chemicals legislation for the benefit of human health and the environment as well as for innovation and competitiveness. | |
Explanation | Use of the information, documents and data from the ECHA website is subject to the terms and conditions of this Legal Notice, and subject to other binding limitations provided for under applicable law, the information, documents and data made available on the ECHA website may be reproduced, distributed and/or used, totally or in part, for non-commercial purposes provided that ECHA is acknowledged as the source: "Source: European Chemicals Agency, http://echa.europa.eu/". Such acknowledgement must be included in each copy of the material. ECHA permits and encourages organisations and individuals to create links to the ECHA website under the following cumulative conditions: Links can only be made to webpages that provide a link to the Legal Notice page. | |
Record name | 1,4,5,8-NAPHTHALENE TETRACARBOXYLIC ACID | |
Source | FDA Global Substance Registration System (GSRS) | |
URL | https://gsrs.ncats.nih.gov/ginas/app/beta/substances/UNB96NX2F8 | |
Description | The FDA Global Substance Registration System (GSRS) enables the efficient and accurate exchange of information on what substances are in regulated products. Instead of relying on names, which vary across regulatory domains, countries, and regions, the GSRS knowledge base makes it possible for substances to be defined by standardized, scientific descriptions. | |
Explanation | Unless otherwise noted, the contents of the FDA website (www.fda.gov), both text and graphics, are not copyrighted. They are in the public domain and may be republished, reprinted and otherwise used freely by anyone without the need to obtain permission from FDA. Credit to the U.S. Food and Drug Administration as the source is appreciated but not required. | |
Synthesis routes and methods I
Procedure details
Synthesis routes and methods II
Procedure details
Retrosynthesis Analysis
AI-Powered Synthesis Planning: Our tool employs the Template_relevance Pistachio, Template_relevance Bkms_metabolic, Template_relevance Pistachio_ringbreaker, Template_relevance Reaxys, Template_relevance Reaxys_biocatalysis model, leveraging a vast database of chemical reactions to predict feasible synthetic routes.
One-Step Synthesis Focus: Specifically designed for one-step synthesis, it provides concise and direct routes for your target compounds, streamlining the synthesis process.
Accurate Predictions: Utilizing the extensive PISTACHIO, BKMS_METABOLIC, PISTACHIO_RINGBREAKER, REAXYS, REAXYS_BIOCATALYSIS database, our tool offers high-accuracy predictions, reflecting the latest in chemical research and data.
Strategy Settings
Precursor scoring | Relevance Heuristic |
---|---|
Min. plausibility | 0.01 |
Model | Template_relevance |
Template Set | Pistachio/Bkms_metabolic/Pistachio_ringbreaker/Reaxys/Reaxys_biocatalysis |
Top-N result to add to graph | 6 |
Feasible Synthetic Routes
Disclaimer and Information on In-Vitro Research Products
Please be aware that all articles and product information presented on BenchChem are intended solely for informational purposes. The products available for purchase on BenchChem are specifically designed for in-vitro studies, which are conducted outside of living organisms. In-vitro studies, derived from the Latin term "in glass," involve experiments performed in controlled laboratory settings using cells or tissues. It is important to note that these products are not categorized as medicines or drugs, and they have not received approval from the FDA for the prevention, treatment, or cure of any medical condition, ailment, or disease. We must emphasize that any form of bodily introduction of these products into humans or animals is strictly prohibited by law. It is essential to adhere to these guidelines to ensure compliance with legal and ethical standards in research and experimentation.