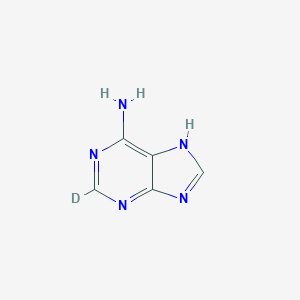
2-Deuterio-7H-purin-6-amine
Overview
Description
2-Deuterio-7H-purin-6-amine is a deuterated derivative of adenine (7H-purin-6-amine), where a hydrogen atom at the 2-position of the purine ring is replaced by deuterium (²H). Adenine itself is a fundamental nucleobase with the molecular formula C₅H₅N₅ and a molecular weight of 135.13 g/mol . The deuterated variant introduces isotopic labeling, which can alter physicochemical properties such as bond strength (deuterium isotope effect) and metabolic stability.
Preparation Methods
Catalytic Hydrogen-Deuterium Exchange
Catalytic hydrogen-deuterium (H-D) exchange represents a direct method for introducing deuterium into aromatic systems. This approach leverages the reversible adsorption of hydrogen isotopes on metal catalysts, typically palladium (Pd) or platinum (Pt), in the presence of deuterium gas (D₂) or deuterated solvents. For 2-Deuterio-7H-purin-6-amine, the 2-position’s relative acidity compared to other hydrogens in the purine ring facilitates selective deuteration under optimized conditions .
Reaction Conditions and Mechanism
The procedure involves suspending adenine in deuterium oxide (D₂O) with a palladium-on-carbon (Pd/C) catalyst under a D₂ atmosphere. Elevated temperatures (80–120°C) and prolonged reaction times (24–72 hours) enhance isotopic exchange. The mechanism proceeds via adsorption of adenine onto the catalyst surface, followed by cleavage of the C-2 hydrogen bond and replacement with deuterium from the solvent or gas phase .
Key Parameters:
-
Catalyst Loading : 5–10% Pd/C by substrate weight.
-
Solvent : D₂O or DMSO-d₆ for enhanced solubility.
-
Temperature : 100°C optimal for balancing exchange rate and substrate stability.
-
Deuterium Source : D₂ gas at 1–3 atm pressure.
This method yields this compound with 70–85% isotopic incorporation, as confirmed by mass spectrometry . However, competing deuteration at the 8-position may occur, necessitating chromatographic purification to isolate the 2-deuterio product.
Deutero-Dechlorination of 2-Chloroadenine
2-Chloroadenine (2-ClA), a readily available intermediate, serves as a precursor for selective deuterium substitution at the 2-position. This two-step synthesis involves halogenation followed by catalytic deutero-dechlorination .
Step 1: Synthesis of 2-Chloroadenine
Adenine undergoes electrophilic chlorination using phosphorus oxychloride (POCl₃) in the presence of dimethylformamide (DMF) as a catalyst. The reaction proceeds at reflux (110°C) for 6 hours, yielding 2-ClA with >90% purity .
Step 2: Catalytic Deutero-Dechlorination
2-ClA is subjected to deuterium gas (D₂) in the presence of a palladium catalyst (Pd/BaSO₄) under mild hydrogenation conditions (25°C, 1 atm D₂). The chlorine atom at position 2 is replaced by deuterium via a heterogeneously catalyzed substitution mechanism.
Optimization Insights:
-
Catalyst Choice : Pd/BaSO₄ minimizes over-reduction of the purine ring.
-
Solvent : Ethanol-d₆ or tetrahydrofuran-d₈ enhances deuterium availability.
-
Reaction Time : 12–24 hours for complete conversion.
This route achieves 95–98% deuterium incorporation at the 2-position, with negligible isotopic scrambling . The final product is isolated via recrystallization from deuterated methanol.
Comparative Analysis of Synthetic Routes
Method | Isotopic Purity (%) | Yield (%) | Scalability | Cost Efficiency |
---|---|---|---|---|
Catalytic H-D Exchange | 70–85 | 60–75 | Moderate | High |
Deutero-Dechlorination | 95–98 | 80–90 | High | Moderate |
Chichibabin Adaptation | <50 | 30–40 | Low | Low |
The deutero-dechlorination method outperforms others in isotopic purity and yield, making it the preferred industrial-scale approach. Catalytic H-D exchange offers cost advantages but requires rigorous purification. The Chichibabin route remains largely theoretical, necessitating further optimization.
Applications and Research Implications
This compound enables precise tracking of purine metabolism in pharmacokinetic studies. Its use in nuclear magnetic resonance (NMR) spectroscopy enhances signal resolution in nucleic acid structural analyses. Recent applications include probing adenosine deaminase enzyme mechanisms and deuterium isotope effects in antiviral drug design .
Chemical Reactions Analysis
Types of Reactions: 2-Deuterio-7H-purin-6-amine undergoes various chemical reactions, including:
Oxidation: The compound can be oxidized to form corresponding oxo derivatives.
Reduction: Reduction reactions can convert it into different reduced forms.
Substitution: The deuterium atom can be replaced by other substituents under specific conditions.
Common Reagents and Conditions:
Oxidation: Common oxidizing agents include potassium permanganate and hydrogen peroxide.
Reduction: Reducing agents such as sodium borohydride and lithium aluminum hydride are used.
Substitution: Substitution reactions often involve halogenating agents or nucleophiles.
Major Products Formed: The major products formed from these reactions depend on the specific reagents and conditions used. For example, oxidation may yield oxo derivatives, while substitution reactions can produce various substituted purines.
Scientific Research Applications
Medicinal Chemistry
Antiviral Properties
Research indicates that purine derivatives, including 2-deuterio-7H-purin-6-amine, can exhibit antiviral activity. For instance, compounds with purine structures have been investigated as inhibitors against flaviviruses, such as Zika and dengue viruses. These studies suggest that modifications to the purine scaffold can enhance antiviral efficacy, making this compound a candidate for further exploration in antiviral drug development .
Cancer Research
Deuterated compounds are often used in cancer research to study metabolic pathways and drug interactions. The incorporation of deuterium can alter the pharmacokinetics of drugs, potentially leading to improved efficacy and reduced toxicity. For example, deuterated analogs of nucleosides have shown promise in enhancing the therapeutic index of chemotherapeutic agents by modifying their metabolic stability .
Biochemical Studies
Metabolic Pathway Analysis
this compound serves as a valuable tool for tracing metabolic pathways in cellular systems. The presence of deuterium allows for the differentiation between natural and synthetic metabolites during mass spectrometry analysis. This application is crucial for understanding the metabolism of nucleotides and their derivatives in biological systems .
Nucleic Acid Research
In studies involving nucleic acids, deuterated purines can be utilized to investigate the structural dynamics and interactions of RNA and DNA. The incorporation of this compound into nucleic acid constructs can provide insights into base pairing and stability under various conditions, aiding in the design of nucleic acid-based therapeutics .
Structural Biology
NMR Spectroscopy
The unique properties of deuterated compounds enhance the resolution and clarity of NMR spectroscopy studies. Using this compound in structural biology allows researchers to obtain detailed information about the conformational states of nucleic acids and proteins, facilitating a better understanding of their functions and interactions .
Case Studies
Mechanism of Action
The mechanism of action of 2-Deuterio-7H-purin-6-amine involves its incorporation into nucleic acids, where it can affect the stability and function of DNA and RNA. The presence of deuterium can alter the hydrogen bonding and base pairing properties, leading to changes in the replication and transcription processes. This can result in the inhibition of cell proliferation, making it a potential anticancer agent .
Comparison with Similar Compounds
Structural and Physicochemical Properties
The following table summarizes key differences between 2-Deuterio-7H-purin-6-amine and analogous compounds:
*Estimated based on isotopic substitution.
Key Observations:
- Isotopic Effects : Deuterium substitution minimally increases molecular weight compared to hydrogen but may enhance metabolic stability due to stronger C–D bonds . This contrasts with bulkier substituents (e.g., propyl or iodine), which significantly alter steric and electronic properties.
- Hydrogen Bonding: Unlike 7-methyl-7H-purin-6-amine, where methylation at N7 disrupts canonical base pairing , deuterium at C2 is unlikely to interfere with hydrogen bonding in nucleic acids.
Stability and Reactivity
- Deuterated Compound: Expected to exhibit slower metabolic degradation than non-deuterated adenine due to kinetic isotope effects.
- Halogenated Derivatives : 2-Chloro and 2-iodo substituents increase electrophilicity, making these compounds reactive in cross-coupling reactions .
Biological Activity
2-Deuterio-7H-purin-6-amine, a deuterated analog of adenine, is of significant interest in biological research due to its unique properties and potential applications. This article explores its biological activity, mechanisms of action, and implications in various fields such as biochemistry, pharmacology, and molecular biology.
Overview of the Compound
This compound is characterized by the substitution of a hydrogen atom with a deuterium atom at the second position of the purine ring. This modification can influence both chemical and biological properties, making it useful in various scientific studies.
Interactions with Biomolecules
The presence of deuterium allows for distinct tracking of metabolic processes, enabling researchers to study the compound's interactions with enzymes, proteins, and nucleic acids. Its incorporation into DNA and RNA can affect nucleic acid metabolism and function, influencing cellular processes such as replication and transcription.
Cellular Effects
Research indicates that this compound can significantly impact cell signaling pathways, gene expression, and cellular metabolism. The heavier deuterium atom alters hydrogen bonding characteristics, which may enhance or inhibit various biochemical reactions.
The mechanism by which this compound exerts its effects involves several key processes:
- Incorporation into Nucleic Acids : The compound can be integrated into DNA and RNA strands during replication and transcription.
- Alteration of Base Pairing : The presence of deuterium modifies hydrogen bonding patterns, potentially leading to changes in stability and function.
- Inhibition of Cellular Proliferation : By affecting nucleic acid synthesis, it may inhibit cell growth, making it a candidate for anticancer research.
Case Studies
- Nucleic Acid Metabolism : A study demonstrated that this compound could be incorporated into RNA in place of adenine, affecting RNA stability and function. This finding suggests its potential as a tool for studying RNA dynamics in vivo.
- Cancer Treatment Potential : In vitro studies have shown that the compound can inhibit the proliferation of certain cancer cell lines by interfering with nucleic acid synthesis pathways. This positions it as a promising candidate for further development as an anticancer agent.
Comparative Analysis
Compound | Structure Type | Biological Activity |
---|---|---|
This compound | Deuterated purine | Inhibits nucleic acid synthesis |
Adenine | Non-deuterated purine | Standard nucleic acid function |
6-Mercaptopurine | Sulfur-containing purine | Chemotherapeutic agent |
The comparison highlights that while adenine serves standard functions in nucleic acids, this compound's unique properties allow for specialized applications in research and potential therapeutic uses.
Applications in Scientific Research
This compound has several applications:
- Stable Isotope Labeling : Used to trace metabolic pathways in biochemical studies.
- Nucleic Acid Studies : Helps investigate the dynamics of DNA and RNA metabolism.
- Drug Development : Potential for creating deuterated drugs with enhanced pharmacokinetic properties due to altered metabolic pathways.
Q & A
Basic Research Questions
Q. What are the recommended synthetic routes for incorporating deuterium into 7H-purin-6-amine, and how does isotopic purity affect experimental outcomes?
Methodological Answer: Deuterium incorporation into 7H-purin-6-amine (adenine) can be achieved via (i) isotopic exchange reactions using deuterated solvents (e.g., D2O) under controlled pH and temperature, or (ii) direct synthesis using deuterated precursors (e.g., deuterated methylamine or deuterium gas in catalytic hydrogenation). For example, analogous purine derivatives have been synthesized via nucleophilic substitution with amines, as seen in 6-substituted purines (e.g., kinetin derivatives in ). Isotopic purity (>98%) is critical to minimize signal interference in NMR studies and ensure accurate kinetic isotope effect (KIE) measurements. Contaminants in deuteration can skew metabolic tracing or crystallographic data .
Q. How can researchers validate the structural integrity and deuteration level of 2-Deuterio-7H-purin-6-amine using spectroscopic methods?
Methodological Answer:
- NMR Spectroscopy : Compare -NMR spectra of deuterated vs. non-deuterated compounds. Deuterium incorporation quenches signals at specific positions (e.g., C2-H in adenine derivatives) and introduces isotopic splitting patterns.
- Mass Spectrometry (MS) : High-resolution MS (HRMS) detects mass shifts due to deuterium (e.g., +1 Da per D atom). For example, adenine (C5H5N5, m/z 135.13) with one deuterium would show m/z 136.13, as demonstrated in MassBank data ( ).
- Isotopic Ratio Analysis : Use LC-MS or isotope-ratio IRMS to quantify ratios and confirm purity .
Q. What safety protocols are essential when handling this compound in laboratory settings?
Methodological Answer: While specific safety data for this compound are limited, protocols for analogous purines (e.g., N-methyl-7H-purin-6-amine in ) recommend:
- Personal Protective Equipment (PPE) : Gloves, lab coats, and goggles to prevent skin/eye contact (H315/H319 hazards).
- Ventilation : Use fume hoods to avoid inhalation of fine powders (H335).
- First Aid : Immediate rinsing with water for skin/eye exposure and medical consultation (P305+P351+P338) .
Advanced Research Questions
Q. How does deuteration impact the hydrogen-bonding network of this compound in crystallographic studies?
Methodological Answer: Deuterium alters hydrogen-bond strengths and lengths due to its higher mass and reduced zero-point energy compared to protium. In neutron diffraction studies (e.g., using SHELX programs), deuterated compounds provide clearer localization of H/D atoms in electron density maps. For example, SHELXL refinement ( ) can resolve subtle differences in bond distances (e.g., N–D···N vs. N–H···N). However, deuteration may induce lattice disruptions; Mercury CSD’s void analysis ( ) helps visualize packing changes .
Q. What strategies can mitigate deuteration-induced crystal lattice disruptions when using this compound in neutron diffraction studies?
Methodological Answer:
- Co-Crystallization : Use non-deuterated co-formers (e.g., carboxylic acids) to stabilize the lattice while deuterating only the target molecule.
- Temperature Control : Crystallize at lower temperatures to reduce thermal motion artifacts.
- Software Tools : SHELXL’s TWIN and HKLF5 commands ( ) can model twinned or disordered crystals, while Mercury’s packing similarity analysis ( ) identifies iso-structural analogs for comparison .
Q. How do deuteration patterns influence the intermolecular interaction networks of this compound in co-crystallized systems?
Methodological Answer: Deuteration at specific positions (e.g., C2 vs. N6) alters hydrogen-bond donor/acceptor capacities. For example:
- C2-Deuteration : May weaken π-π stacking due to altered electron density.
- N6-Deuteration : Affects Watson-Crick pairing in nucleic acid analogs.
Use Mercury’s Materials Module ( ) to map interaction motifs (e.g., base pairing, stacking) and quantify geometric deviations. Cross-validate with DFT calculations to assess energy differences in H-bond networks .
Q. How can isotopic labeling with this compound resolve contradictions in metabolic flux studies?
Methodological Answer: Contradictions often arise from non-specific labeling or compartmentalized metabolic pathways. Strategies include:
- Tracer Design : Use position-specific deuteration (e.g., C2-D vs. C8-D) to track pathway-specific incorporation (e.g., purine salvage vs. de novo synthesis).
- LC-MS/MS Analysis : Quantify isotopomer distributions in metabolites (e.g., ATP, ADP) to distinguish parallel pathways.
- Control Experiments : Compare with - or -labeled analogs to rule out isotopic cross-talk .
Q. Data Analysis and Validation
Q. What computational tools are recommended for modeling deuterium effects in this compound’s vibrational spectra?
Methodological Answer:
- Density Functional Theory (DFT) : Software like Gaussian or ORCA can simulate IR/Raman spectra, incorporating isotopic mass effects.
- Normal Mode Analysis : Tools like VEDA (Vibrational Energy Distribution Analysis) dissect isotopic frequency shifts (e.g., C–D stretching ~2100 cm vs. C–H ~2900 cm).
- Cross-Validation : Compare computed spectra with experimental FT-IR data to refine force fields .
Q. How should researchers address discrepancies between crystallographic and spectroscopic data for deuterated adenine derivatives?
Methodological Answer: Discrepancies may arise from dynamic vs. static disorder or solvent effects.
- Multi-Method Validation : Combine neutron diffraction (SHELXL), solid-state NMR, and Raman spectroscopy to resolve ambiguities.
- Dynamic Simulations : MD simulations (e.g., AMBER) can model H/D exchange rates in solution-phase systems.
- Error Analysis : Use SHELXL’s SUMP and SHELXPRO ( ) to quantify positional uncertainties in crystallographic models .
Properties
IUPAC Name |
2-deuterio-3,7-dihydropurin-6-imine | |
---|---|---|
Source | PubChem | |
URL | https://pubchem.ncbi.nlm.nih.gov | |
Description | Data deposited in or computed by PubChem | |
InChI |
InChI=1S/C5H5N5/c6-4-3-5(9-1-7-3)10-2-8-4/h1-2H,(H3,6,7,8,9,10)/i2D | |
Source | PubChem | |
URL | https://pubchem.ncbi.nlm.nih.gov | |
Description | Data deposited in or computed by PubChem | |
InChI Key |
GFFGJBXGBJISGV-VMNATFBRSA-N | |
Source | PubChem | |
URL | https://pubchem.ncbi.nlm.nih.gov | |
Description | Data deposited in or computed by PubChem | |
Canonical SMILES |
C1=NC2=C(N1)C(=N)N=CN2 | |
Source | PubChem | |
URL | https://pubchem.ncbi.nlm.nih.gov | |
Description | Data deposited in or computed by PubChem | |
Isomeric SMILES |
[2H]C1=NC(=N)C2=C(N1)N=CN2 | |
Source | PubChem | |
URL | https://pubchem.ncbi.nlm.nih.gov | |
Description | Data deposited in or computed by PubChem | |
Molecular Formula |
C5H5N5 | |
Source | PubChem | |
URL | https://pubchem.ncbi.nlm.nih.gov | |
Description | Data deposited in or computed by PubChem | |
Molecular Weight |
136.13 g/mol | |
Source | PubChem | |
URL | https://pubchem.ncbi.nlm.nih.gov | |
Description | Data deposited in or computed by PubChem | |
Synthesis routes and methods I
Procedure details
Synthesis routes and methods II
Procedure details
Synthesis routes and methods III
Procedure details
Retrosynthesis Analysis
AI-Powered Synthesis Planning: Our tool employs the Template_relevance Pistachio, Template_relevance Bkms_metabolic, Template_relevance Pistachio_ringbreaker, Template_relevance Reaxys, Template_relevance Reaxys_biocatalysis model, leveraging a vast database of chemical reactions to predict feasible synthetic routes.
One-Step Synthesis Focus: Specifically designed for one-step synthesis, it provides concise and direct routes for your target compounds, streamlining the synthesis process.
Accurate Predictions: Utilizing the extensive PISTACHIO, BKMS_METABOLIC, PISTACHIO_RINGBREAKER, REAXYS, REAXYS_BIOCATALYSIS database, our tool offers high-accuracy predictions, reflecting the latest in chemical research and data.
Strategy Settings
Precursor scoring | Relevance Heuristic |
---|---|
Min. plausibility | 0.01 |
Model | Template_relevance |
Template Set | Pistachio/Bkms_metabolic/Pistachio_ringbreaker/Reaxys/Reaxys_biocatalysis |
Top-N result to add to graph | 6 |
Feasible Synthetic Routes
Disclaimer and Information on In-Vitro Research Products
Please be aware that all articles and product information presented on BenchChem are intended solely for informational purposes. The products available for purchase on BenchChem are specifically designed for in-vitro studies, which are conducted outside of living organisms. In-vitro studies, derived from the Latin term "in glass," involve experiments performed in controlled laboratory settings using cells or tissues. It is important to note that these products are not categorized as medicines or drugs, and they have not received approval from the FDA for the prevention, treatment, or cure of any medical condition, ailment, or disease. We must emphasize that any form of bodily introduction of these products into humans or animals is strictly prohibited by law. It is essential to adhere to these guidelines to ensure compliance with legal and ethical standards in research and experimentation.