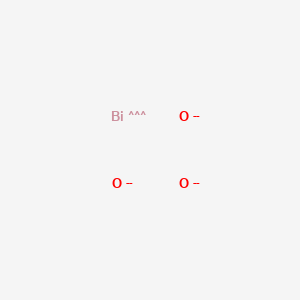
Bismuth oxide (Bi2O3)
- Click on QUICK INQUIRY to receive a quote from our team of experts.
- With the quality product at a COMPETITIVE price, you can focus more on your research.
Overview
Description
Bismuth oxide (Bi2O3) is an inorganic compound that has garnered significant attention in scientific and industrial research due to its unique properties. It is characterized by its yellow color and exists in several polymorphic forms, including alpha (α), beta (β), gamma (γ), and delta (δ) phases. The alpha phase is stable at room temperature and has a monoclinic crystal structure, while the beta phase is stable at higher temperatures and has a cubic fluorite-like structure .
Preparation Methods
Bismuth oxide can be synthesized through various methods, each yielding different levels of purity and crystalline structures. The primary methods include:
Thermal Decomposition: Bismuth (III) nitrate is gently heated until it decomposes into bismuth oxide, nitrogen dioxide, and oxygen. The chemical equation for this reaction is[ 2Bi(NO_3)_3 \rightarrow 2Bi_2O_3 + 6NO_2 + O_2 ]
Precipitation Method: Bismuth oxide can also be obtained through the precipitation of bismuth salts using strong alkalis such as sodium hydroxide.
Solution Combustion Synthesis: This method involves the use of a fuel, such as sucrose, to rapidly produce bismuth oxide through an exothermic reaction.
Chemical Reactions Analysis
Bismuth oxide undergoes various chemical reactions, including:
Reduction: Bismuth oxide can be reduced to metallic bismuth using reducing agents such as ethanol.
Photocatalytic Reactions: Bismuth oxide is a promising photocatalyst for the decomposition of pollutants under visible and ultraviolet light.
Scientific Research Applications
Bismuth oxide has a wide range of applications in various fields:
Mechanism of Action
The mechanism by which bismuth oxide exerts its effects varies depending on its application:
Photocatalysis: Bismuth oxide extends its photoresponse to the visible light domain, making it effective in degrading pollutants.
Biomedical Applications: In cancer treatment, bismuth oxide nanoparticles enhance imaging and therapeutic efficacy by targeting tumor cells and providing contrast in imaging techniques.
Comparison with Similar Compounds
Bismuth oxide can be compared with other bismuth compounds, such as:
Bismuth Oxyhalides (BiOX): These compounds have similar photocatalytic properties but differ in their bandgap and structural properties.
Bismuth Vanadate (BiVO4): Known for its photocatalytic activity, bismuth vanadate has a narrower bandgap compared to bismuth oxide.
Bismuth Molybdate (Bi2MoO6): This compound is also used in photocatalysis and has different structural and electronic properties compared to bismuth oxide.
Bismuth oxide stands out due to its high thermal stability, unique crystalline phases, and versatile applications across various fields.
Properties
Molecular Formula |
BiO3-6 |
---|---|
Molecular Weight |
256.979 g/mol |
InChI |
InChI=1S/Bi.3O/q;3*-2 |
InChI Key |
MEPZNTYFGPFXIA-UHFFFAOYSA-N |
Canonical SMILES |
[O-2].[O-2].[O-2].[Bi] |
Origin of Product |
United States |
Disclaimer and Information on In-Vitro Research Products
Please be aware that all articles and product information presented on BenchChem are intended solely for informational purposes. The products available for purchase on BenchChem are specifically designed for in-vitro studies, which are conducted outside of living organisms. In-vitro studies, derived from the Latin term "in glass," involve experiments performed in controlled laboratory settings using cells or tissues. It is important to note that these products are not categorized as medicines or drugs, and they have not received approval from the FDA for the prevention, treatment, or cure of any medical condition, ailment, or disease. We must emphasize that any form of bodily introduction of these products into humans or animals is strictly prohibited by law. It is essential to adhere to these guidelines to ensure compliance with legal and ethical standards in research and experimentation.