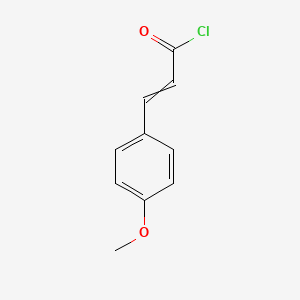
4-Methoxycinnamic acid chloride
Overview
Description
4-Methoxycinnamic acid chloride (CAS 34446-64-5), also known as p-methoxycinnamoyl chloride, is a reactive acyl chloride derivative of 4-methoxycinnamic acid. Its molecular formula is C₁₀H₉ClO₂, featuring a methoxy (-OCH₃) group at the para position of the cinnamic acid backbone . This compound is widely utilized in organic synthesis, particularly in the preparation of neoflavonoids, phthalimide derivatives, and other bioactive molecules .
Synthesis: this compound is typically synthesized via the reaction of 4-methoxycinnamic acid with oxalyl chloride in carbon disulfide, followed by purification using column chromatography. Yields vary depending on conditions; for example, a synthesis reported an 8.47% yield when reacting with 3-pentadecylphenol and aluminium trichloride .
Preparation Methods
Synthetic Routes and Reaction Conditions: 4-Methoxycinnamic acid chloride can be synthesized through the reaction of paramethoxycinnamic acid with thionyl chloride (SOCl2) or oxalyl chloride (COCl)2. The reaction typically involves refluxing the mixture to ensure complete conversion of the carboxylic acid to the acyl chloride. The reaction can be represented as follows:
C10H10O3 (Paramethoxycinnamic acid)+SOCl2→C10H9ClO2 (Paramethoxycinnamoyl chloride)+SO2+HCl
Industrial Production Methods: In industrial settings, the production of paramethoxycinnamoyl chloride follows similar synthetic routes but on a larger scale. The use of continuous flow reactors and optimized reaction conditions ensures high yield and purity of the product. The reaction conditions are carefully controlled to avoid side reactions and degradation of the product.
Chemical Reactions Analysis
Types of Reactions: 4-Methoxycinnamic acid chloride undergoes various chemical reactions, including:
Nucleophilic Substitution: It reacts with nucleophiles such as amines, alcohols, and thiols to form corresponding amides, esters, and thioesters.
Hydrolysis: In the presence of water, it hydrolyzes to form paramethoxycinnamic acid and hydrochloric acid.
Reduction: It can be reduced to paramethoxycinnamyl alcohol using reducing agents like lithium aluminum hydride (LiAlH4).
Common Reagents and Conditions:
Nucleophilic Substitution: Reagents like amines, alcohols, and thiols in the presence of a base (e.g., pyridine) are commonly used.
Hydrolysis: Water or aqueous base (e.g., NaOH) can be used.
Reduction: Lithium aluminum hydride (LiAlH4) or sodium borohydride (NaBH4) are typical reducing agents.
Major Products:
Amides, esters, and thioesters: from nucleophilic substitution.
Paramethoxycinnamic acid: from hydrolysis.
Paramethoxycinnamyl alcohol: from reduction.
Scientific Research Applications
Chemistry: 4-Methoxycinnamic acid chloride is used as an intermediate in the synthesis of various organic compounds, including pharmaceuticals, agrochemicals, and dyes.
Biology and Medicine: It is used in the synthesis of bioactive molecules with potential antimicrobial, antifungal, and anticancer properties. Its derivatives are studied for their ability to inhibit the growth of pathogenic microorganisms and cancer cells .
Industry: In the industrial sector, paramethoxycinnamoyl chloride is used in the production of UV-absorbing agents, which are incorporated into sunscreens and other cosmetic products to protect the skin from harmful UV radiation .
Mechanism of Action
The mechanism of action of paramethoxycinnamoyl chloride and its derivatives involves interaction with cellular components. For instance, some derivatives interact with the ergosterol present in fungal cell membranes, disrupting their integrity and leading to cell death . In antibacterial applications, it targets specific bacterial enzymes, inhibiting their function and leading to bacterial cell death .
Comparison with Similar Compounds
Structural and Functional Group Variations
Key structural analogs include cinnamic acid derivatives with varying substituents on the aromatic ring. These differences influence reactivity, stability, and biological activity:
Key Research Findings
- Substituent Effects: Methoxy groups enhance electron density, improving resonance stabilization in intermediates. However, additional substituents (e.g., 3,4-dimethoxy) can sterically hinder reactions, reducing yields compared to monosubstituted analogs .
- Yield Optimization: Reactions involving this compound often require controlled conditions (e.g., ice baths, anhydrous solvents) to mitigate hydrolysis, a challenge less pronounced in methyl- or chloro-substituted derivatives .
Q & A
Basic Research Questions
Q. What are the optimal reaction conditions for synthesizing 4-methoxycinnamic acid chloride from its acid precursor?
The synthesis typically involves converting 4-methoxycinnamic acid to its chloride derivative using reagents like thionyl chloride (SOCl₂) or oxalyl chloride under anhydrous conditions. For example, the Knoevenagel condensation method has been employed to synthesize (E)-4-methoxycinnamic acid, which can then undergo chlorination. Key factors include maintaining a moisture-free environment, controlling reaction temperature (e.g., reflux in dry dichloromethane), and using catalytic dimethylformamide (DMF) to enhance reactivity. Post-synthesis purification via vacuum distillation or recrystallization ensures high purity .
Q. Which spectroscopic techniques are most effective for characterizing this compound?
Infrared (IR) spectroscopy is critical for identifying functional groups, such as the C=O stretch of the acyl chloride (~1800 cm⁻¹) and the methoxy group (~1250 cm⁻¹). Nuclear magnetic resonance (NMR) spectroscopy (¹H and ¹³C) provides structural details, including the trans-configuration of the double bond (J ≈ 16 Hz for vicinal protons) and aromatic substitution patterns. Mass spectrometry (MS) confirms molecular weight (e.g., m/z 196 for [M+H]⁺). Thermal analysis (e.g., DSC) can also monitor phase transitions, as seen in its crystalline-to-isotropic transitions at ~190°C .
Q. What safety protocols are essential when handling this compound in the laboratory?
Due to its irritant properties (skin, eye, and respiratory tract), use personal protective equipment (PPE), including nitrile gloves, goggles, and a lab coat. Work in a fume hood to avoid inhalation. In case of exposure, rinse affected areas with copious water and seek medical attention. Store the compound in a cool, dry place, away from moisture and incompatible substances (e.g., bases, oxidizing agents) .
Advanced Research Questions
Q. How can density functional theory (DFT) predict the reactivity of this compound in halogenation reactions?
Computational studies using DFT and MP2 methods have shown that reactions with Cl₂ or HOCl are thermodynamically favorable. For instance, the electron-withdrawing methoxy group stabilizes intermediates via resonance, lowering activation barriers. Researchers can model reaction pathways, calculate Gibbs free energy changes, and predict regioselectivity by analyzing frontier molecular orbitals (FMOs) and electrostatic potential maps .
Q. What role does this compound play in synthesizing UV-protective materials?
The chloride derivative serves as a precursor for esters (e.g., ethyl 4-methoxycinnamate) used in UV filters. These esters absorb UV-A/UV-B radiation by conjugating the π-electrons of the cinnamoyl group. Experimental protocols involve coupling the chloride with alcohols (e.g., ethanol) in the presence of a base (e.g., triethylamine) to minimize side reactions. The resulting compounds are incorporated into polymers or coatings, with efficacy validated via UV-Vis spectroscopy and photostability assays .
Q. How can isotopic labeling of this compound elucidate metabolic pathways in plant cell cultures?
Feeding ¹³C- or ¹⁴C-labeled this compound to Vanilla planifolia cell suspensions allows tracking its conversion into metabolites like 4-hydroxybenzoic acid. Liquid chromatography-mass spectrometry (LC-MS) and radioisotope tracing quantify metabolic flux. Enzymatic studies (e.g., using phenylpropanoid pathway inhibitors) can identify rate-limiting steps, such as O-demethylation by cytochrome P450 enzymes .
Q. How do structural modifications of this compound influence its antibacterial activity?
Modifying the acyl chloride group to amides or hydrazides enhances bioactivity. For example, coupling with amines generates derivatives with improved membrane permeability. Minimum inhibitory concentration (MIC) assays against Gram-positive/negative bacteria, combined with molecular docking studies, reveal interactions with bacterial enzymes (e.g., DNA gyrase). Structure-activity relationship (SAR) models optimize substituent effects on potency .
Q. Methodological Considerations
- Data Contradiction Analysis : Discrepancies in reported melting points (e.g., 170–173°C vs. higher values) may arise from polymorphic forms or impurities. Use differential scanning calorimetry (DSC) to verify phase transitions and high-performance liquid chromatography (HPLC) to assess purity .
- Experimental Design : For kinetic studies of halogenation, employ stopped-flow techniques with UV-Vis monitoring to capture transient intermediates. Compare computational predictions with experimental rate constants .
Properties
Molecular Formula |
C10H9ClO2 |
---|---|
Molecular Weight |
196.63 g/mol |
IUPAC Name |
3-(4-methoxyphenyl)prop-2-enoyl chloride |
InChI |
InChI=1S/C10H9ClO2/c1-13-9-5-2-8(3-6-9)4-7-10(11)12/h2-7H,1H3 |
InChI Key |
CGOJOQBYEAVATL-UHFFFAOYSA-N |
Canonical SMILES |
COC1=CC=C(C=C1)C=CC(=O)Cl |
Origin of Product |
United States |
Synthesis routes and methods I
Procedure details
Synthesis routes and methods II
Procedure details
Synthesis routes and methods III
Procedure details
Disclaimer and Information on In-Vitro Research Products
Please be aware that all articles and product information presented on BenchChem are intended solely for informational purposes. The products available for purchase on BenchChem are specifically designed for in-vitro studies, which are conducted outside of living organisms. In-vitro studies, derived from the Latin term "in glass," involve experiments performed in controlled laboratory settings using cells or tissues. It is important to note that these products are not categorized as medicines or drugs, and they have not received approval from the FDA for the prevention, treatment, or cure of any medical condition, ailment, or disease. We must emphasize that any form of bodily introduction of these products into humans or animals is strictly prohibited by law. It is essential to adhere to these guidelines to ensure compliance with legal and ethical standards in research and experimentation.