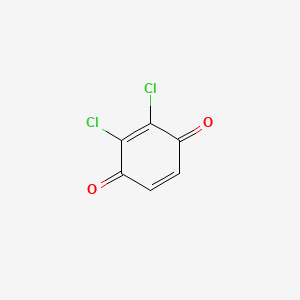
2,3-Dichloro-1,4-benzoquinone
Overview
Description
2,3-Dichloro-1,4-benzoquinone (2,3-DCBQ, CAS 84-58-2) is a chlorinated derivative of 1,4-benzoquinone, characterized by two chlorine substituents at the 2- and 3-positions of the benzene ring. It is a yellow to dark purple crystalline solid, soluble in organic solvents like methanol and benzene but insoluble in water . This compound is synthetically versatile, serving as a precursor in organic reactions such as nucleophilic substitutions and Michael additions. Notably, 2,3-DCBQ derivatives, like 2,3-dichloro-5,6-dicyano-1,4-benzoquinone, are used in spectrophotometric determinations due to their electron-accepting properties . While its direct biological activity is less documented compared to naphthoquinone analogs, 2,3-DCBQ plays a role in detoxification pathways, transforming into less toxic metabolites like 2,3-dichloro-4-methoxyphenol under specific conditions .
Q & A
Basic Research Questions
Q. What analytical techniques are recommended for confirming the structural integrity of 2,3-Dichloro-1,4-benzoquinone?
Methodological Answer: Structural confirmation requires a combination of techniques:
- X-ray crystallography : Resolve molecular geometry and intermolecular interactions (e.g., π-π stacking in charge-transfer complexes) .
- NMR spectroscopy : Identify substitution patterns (e.g., chlorine positions) and monitor reaction intermediates. For example, H NMR distinguishes hydroquinone and quinone forms .
- LC-MS : Track reaction pathways (e.g., nucleophilic substitution) by identifying molecular ion peaks and fragmentation patterns .
- IR spectroscopy : Detect functional groups (e.g., C=O stretching at ~1650 cm) .
Q. How can researchers determine the electrochemical properties of this compound in non-aqueous solvents?
Methodological Answer: Use single potential step chronoamperometry to measure diffusion coefficients () in solvents like acetonitrile. Key steps:
Prepare a three-electrode system (glassy carbon working electrode, Pt counter electrode, Ag/Ag reference).
Record current-time transients under controlled potential.
Apply the Cottrell equation: , where = electrode area, = concentration .
Data Example :
Solvent | Diffusion Coefficient (, cm/s) | Reference |
---|---|---|
Acetonitrile |
Advanced Research Questions
Q. How can computational models resolve contradictions in the oxidative reactivity of this compound?
Methodological Answer: Discrepancies in autoxidation rates (e.g., unexpected fast reactivity despite high redox potential) require DFT calculations to evaluate:
- Charge-transfer complex stability : Hydride transfer barriers and carbocation intermediate stability .
- Secondary orbital interactions : Overlap between substrate π orbitals and the quinone’s LUMO .
- Linear free energy relationships (LFER) : Correlate substituent electronic effects (Hammett σ values) with reaction rates .
Q. What strategies optimize the synthesis of charge-transfer complexes using this compound?
Methodological Answer: Design donor-acceptor systems with controlled stoichiometry:
Co-crystallization : Combine hydroquinone (donor) and quinone (acceptor) in a 1:1 molar ratio in methanol/water .
Stacking geometry : Ensure parallel molecular planes (tilt <2°) for π-π interactions (centroid distance: 3.5–3.9 Å) .
Hydrogen bonding : Stabilize networks via O–H···O interactions (e.g., water-mediated bridges) .
Q. How do substituents influence the antitumor activity of this compound derivatives?
Methodological Answer: Evaluate structure-activity relationships (SAR) through:
- In vivo testing : Inject derivatives into mice with ascitic sarcoma 180; measure tumor inhibition rates .
- Mechanistic assays :
Derivative (R Group) | Tumor Inhibition (%) | Hemolysis Threshold (mM) |
---|---|---|
R = H | 44 | 0.15 |
R = Cl | 68 | 0.08 |
R = NH | 52 | 0.12 |
Source: |
Q. Data Contradiction Analysis
Q. Why do some nucleophilic substitutions with this compound yield monosubstituted products instead of cyclized derivatives?
Methodological Answer: Contradictions arise from reaction conditions:
- Base selection : Triethylamine promotes monosubstitution, while stronger bases (e.g., KOtBu) enable cyclization via dianion intermediates .
- Steric effects : Bulky nucleophiles (e.g., pyrazole) hinder second substitution .
- Temperature : Heating (80°C) favors cyclization by overcoming kinetic barriers .
Q. How can researchers mitigate variability in this compound’s photochemical behavior?
Methodological Answer: Address light-induced variability via:
- Wavelength control : Use UV filters (e.g., 365 nm) to isolate specific excitation pathways .
- Quenching studies : Add radical scavengers (e.g., TEMPO) to identify reactive intermediates .
- Kinetic modeling : Fit time-resolved UV-vis data to pseudo-first-order rate equations .
Q. Method Development
Q. What advanced oxidation processes (AOPs) effectively degrade this compound in water?
Methodological Answer: Optimize AOPs using response surface methodology (RSM):
UV/HO : Vary HO concentration (0.1–1.0 mM) and UV intensity (10–50 mW/cm) .
Ozonation : Monitor ozone dosage (0.5–2.0 mg/L) and pH (3–9) .
Kinetic analysis : Use pseudo-second-order models to fit degradation curves () .
Comparison with Similar Compounds
Comparison with Similar Chlorinated Benzoquinones
Structural and Physical Properties
The reactivity and applications of chlorinated benzoquinones depend on the number and positions of chlorine atoms. Key analogs include:
Reactivity and Chemical Behavior
- Chlorination Degree : Higher chlorination increases electrophilicity and reaction diversity. TCBQ undergoes multi-site substitutions (e.g., with thiols or amines), enabling cross-linking reactions, whereas dichloro analogs exhibit selective reactivity .
- Substituent Position :
- 2,3-DCBQ : Chlorines at adjacent positions enhance electron-withdrawing effects, facilitating nucleophilic attacks at the 5- and 6-positions .
- 2,5-DCBQ : Symmetrical substitution reduces steric hindrance, favoring decomposition into reactive intermediates in aqueous media .
- 2,6-DCBQ : Chlorines at para positions increase stability, making it a persistent DBP with environmental toxicity .
Properties
CAS No. |
26912-70-9 |
---|---|
Molecular Formula |
C6H2Cl2O2 |
Molecular Weight |
176.98 g/mol |
IUPAC Name |
2,3-dichlorocyclohexa-2,5-diene-1,4-dione |
InChI |
InChI=1S/C6H2Cl2O2/c7-5-3(9)1-2-4(10)6(5)8/h1-2H |
InChI Key |
USAYMJGCALIGIG-UHFFFAOYSA-N |
Canonical SMILES |
C1=CC(=O)C(=C(C1=O)Cl)Cl |
Origin of Product |
United States |
Synthesis routes and methods I
Procedure details
Synthesis routes and methods II
Procedure details
Disclaimer and Information on In-Vitro Research Products
Please be aware that all articles and product information presented on BenchChem are intended solely for informational purposes. The products available for purchase on BenchChem are specifically designed for in-vitro studies, which are conducted outside of living organisms. In-vitro studies, derived from the Latin term "in glass," involve experiments performed in controlled laboratory settings using cells or tissues. It is important to note that these products are not categorized as medicines or drugs, and they have not received approval from the FDA for the prevention, treatment, or cure of any medical condition, ailment, or disease. We must emphasize that any form of bodily introduction of these products into humans or animals is strictly prohibited by law. It is essential to adhere to these guidelines to ensure compliance with legal and ethical standards in research and experimentation.