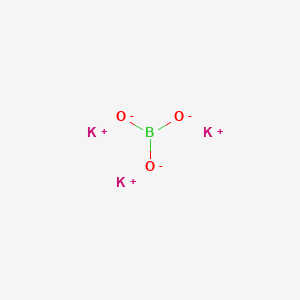
Potassium borate
Overview
Description
Potassium borate is a chemical compound with the formula K₂B₄O₇. It is a salt derived from boric acid and potassium. This compound falls under the category of borate minerals, which have been utilized for thousands of years in various applications, ranging from pottery to flame retardants. This compound is typically a white, crystalline solid that is odorless and has a slightly salty, alkaline taste. It is soluble in water, which makes it advantageous for various applications where it needs to be mixed with liquids .
Preparation Methods
Synthetic Routes and Reaction Conditions: The production of potassium borate typically involves the reaction of borax (sodium borate) with potassium salts. The process begins with the dissolution of borax in water, followed by the addition of a potassium salt such as potassium chloride or potassium carbonate. This reaction results in the precipitation of this compound, which can then be isolated and purified .
Industrial Production Methods: Industrial synthesis of this compound may involve other methods and processes tailored to the specific needs of the application. For example, hydrothermal synthesis from various boron minerals such as boric acid, boron trioxide, and sodium borate under controlled temperature and time conditions can yield this compound .
Chemical Reactions Analysis
Types of Reactions: Potassium borate undergoes various chemical reactions, including:
Oxidation and Reduction: this compound can participate in redox reactions, although specific examples are less common.
Substitution: It can undergo substitution reactions where potassium ions are replaced by other cations.
Common Reagents and Conditions:
Oxidation: Involves oxidizing agents like hydrogen peroxide.
Reduction: Involves reducing agents like sodium borohydride.
Substitution: Involves reagents like sodium chloride or calcium chloride.
Major Products Formed:
Oxidation: Formation of higher oxidation state borates.
Reduction: Formation of lower oxidation state borates.
Substitution: Formation of mixed metal borates.
Scientific Research Applications
Mechanism of Action
The mechanism by which potassium borate exerts its effects involves its interaction with various molecular targets and pathways:
Ion Transport: this compound glass has potential as an ion transport material.
Flame Retardancy: In the event of a fire, this compound reacts to form a glassy layer on the material’s surface, reducing the material’s contribution to the spread of fire.
Anti-fungal and Insecticidal Properties: this compound disrupts the cellular processes of fungi and insects, leading to their elimination.
Comparison with Similar Compounds
Sodium Borate (Borax): Similar in structure and properties but contains sodium instead of potassium.
Calcium Borate: Contains calcium and is used in similar applications such as ceramics and glass production.
Magnesium Borate: Contains magnesium and is used in flame retardants and as a lubricant additive.
Uniqueness of Potassium Borate: this compound is unique due to its specific ionic composition, which imparts distinct properties such as higher solubility in water and specific ion transport characteristics. Its ability to act as a micronutrient fertilizer and its flame-retardant properties make it particularly valuable in agriculture and fire safety applications .
Properties
IUPAC Name |
tripotassium;borate | |
---|---|---|
Source | PubChem | |
URL | https://pubchem.ncbi.nlm.nih.gov | |
Description | Data deposited in or computed by PubChem | |
InChI |
InChI=1S/BO3.3K/c2-1(3)4;;;/q-3;3*+1 | |
Source | PubChem | |
URL | https://pubchem.ncbi.nlm.nih.gov | |
Description | Data deposited in or computed by PubChem | |
InChI Key |
WUUHFRRPHJEEKV-UHFFFAOYSA-N | |
Source | PubChem | |
URL | https://pubchem.ncbi.nlm.nih.gov | |
Description | Data deposited in or computed by PubChem | |
Canonical SMILES |
B([O-])([O-])[O-].[K+].[K+].[K+] | |
Source | PubChem | |
URL | https://pubchem.ncbi.nlm.nih.gov | |
Description | Data deposited in or computed by PubChem | |
Molecular Formula |
BK3O3 | |
Source | PubChem | |
URL | https://pubchem.ncbi.nlm.nih.gov | |
Description | Data deposited in or computed by PubChem | |
DSSTOX Substance ID |
DTXSID3049750 | |
Record name | Potassium borate | |
Source | EPA DSSTox | |
URL | https://comptox.epa.gov/dashboard/DTXSID3049750 | |
Description | DSSTox provides a high quality public chemistry resource for supporting improved predictive toxicology. | |
Molecular Weight |
176.11 g/mol | |
Source | PubChem | |
URL | https://pubchem.ncbi.nlm.nih.gov | |
Description | Data deposited in or computed by PubChem | |
Physical Description |
Liquid | |
Record name | Boric acid, potassium salt | |
Source | EPA Chemicals under the TSCA | |
URL | https://www.epa.gov/chemicals-under-tsca | |
Description | EPA Chemicals under the Toxic Substances Control Act (TSCA) collection contains information on chemicals and their regulations under TSCA, including non-confidential content from the TSCA Chemical Substance Inventory and Chemical Data Reporting. | |
CAS No. |
12712-38-8 | |
Record name | Boric acid, potassium salt | |
Source | ChemIDplus | |
URL | https://pubchem.ncbi.nlm.nih.gov/substance/?source=chemidplus&sourceid=0012712388 | |
Description | ChemIDplus is a free, web search system that provides access to the structure and nomenclature authority files used for the identification of chemical substances cited in National Library of Medicine (NLM) databases, including the TOXNET system. | |
Record name | Boric acid, potassium salt | |
Source | EPA Chemicals under the TSCA | |
URL | https://www.epa.gov/chemicals-under-tsca | |
Description | EPA Chemicals under the Toxic Substances Control Act (TSCA) collection contains information on chemicals and their regulations under TSCA, including non-confidential content from the TSCA Chemical Substance Inventory and Chemical Data Reporting. | |
Record name | Potassium borate | |
Source | EPA DSSTox | |
URL | https://comptox.epa.gov/dashboard/DTXSID3049750 | |
Description | DSSTox provides a high quality public chemistry resource for supporting improved predictive toxicology. | |
Record name | Boric acid, potassium salt | |
Source | European Chemicals Agency (ECHA) | |
URL | https://echa.europa.eu/substance-information/-/substanceinfo/100.113.100 | |
Description | The European Chemicals Agency (ECHA) is an agency of the European Union which is the driving force among regulatory authorities in implementing the EU's groundbreaking chemicals legislation for the benefit of human health and the environment as well as for innovation and competitiveness. | |
Explanation | Use of the information, documents and data from the ECHA website is subject to the terms and conditions of this Legal Notice, and subject to other binding limitations provided for under applicable law, the information, documents and data made available on the ECHA website may be reproduced, distributed and/or used, totally or in part, for non-commercial purposes provided that ECHA is acknowledged as the source: "Source: European Chemicals Agency, http://echa.europa.eu/". Such acknowledgement must be included in each copy of the material. ECHA permits and encourages organisations and individuals to create links to the ECHA website under the following cumulative conditions: Links can only be made to webpages that provide a link to the Legal Notice page. | |
Synthesis routes and methods
Procedure details
Disclaimer and Information on In-Vitro Research Products
Please be aware that all articles and product information presented on BenchChem are intended solely for informational purposes. The products available for purchase on BenchChem are specifically designed for in-vitro studies, which are conducted outside of living organisms. In-vitro studies, derived from the Latin term "in glass," involve experiments performed in controlled laboratory settings using cells or tissues. It is important to note that these products are not categorized as medicines or drugs, and they have not received approval from the FDA for the prevention, treatment, or cure of any medical condition, ailment, or disease. We must emphasize that any form of bodily introduction of these products into humans or animals is strictly prohibited by law. It is essential to adhere to these guidelines to ensure compliance with legal and ethical standards in research and experimentation.