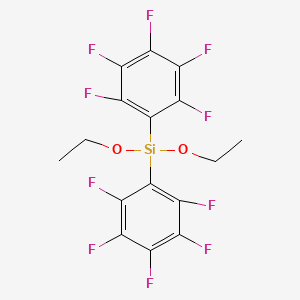
Dimethoxybis(pentafluorophenyl)silane
Overview
Description
Dimethoxybis(pentafluorophenyl)silane: is an organosilicon compound characterized by the presence of two pentafluorophenyl groups and two ethoxy groups attached to a silicon atom. This compound is known for its unique chemical properties, which make it valuable in various scientific and industrial applications.
Preparation Methods
Synthetic Routes and Reaction Conditions: The synthesis of bis(pentafluorophenyl)diethoxysilane typically involves the reaction of pentafluorophenyl lithium with silicon tetrachloride, followed by the addition of ethanol. The reaction is carried out under an inert atmosphere to prevent moisture and oxygen from interfering with the process. The general reaction scheme is as follows:
-
Formation of Pentafluorophenyl Lithium:
C6F5Br+2Li→C6F5Li+LiBr
-
Reaction with Silicon Tetrachloride:
2C6F5Li+SiCl4→(C6F5)2SiCl2+2LiCl
-
Addition of Ethanol:
(C6F5)2SiCl2+2C2H5OH→(C6F5)2Si(OEt)2+2HCl
Industrial Production Methods: Industrial production of bis(pentafluorophenyl)diethoxysilane follows similar synthetic routes but on a larger scale. The process involves careful control of reaction conditions, including temperature, pressure, and the use of high-purity reagents to ensure the quality and yield of the final product.
Chemical Reactions Analysis
Types of Reactions: Dimethoxybis(pentafluorophenyl)silane undergoes various chemical reactions, including:
-
Substitution Reactions:
- Reaction with nucleophiles such as amines or alcohols to replace the ethoxy groups.
- Example: Reaction with an amine to form a silazane derivative.
-
Hydrolysis:
- Reaction with water to form silanols and pentafluorophenol.
- Example: Hydrolysis in the presence of moisture.
-
Cross-Coupling Reactions:
- Palladium-catalyzed cross-coupling reactions with aryl halides.
- Example: Formation of polyfluorophenyl-substituted compounds.
Common Reagents and Conditions:
Nucleophiles: Amines, alcohols.
Catalysts: Palladium catalysts for cross-coupling reactions.
Solvents: Organic solvents such as toluene or THF.
Conditions: Inert atmosphere, controlled temperature.
Major Products:
- Silazane derivatives.
- Silanols.
- Polyfluorophenyl-substituted compounds.
Scientific Research Applications
Chemistry:
- Used as a precursor in the synthesis of advanced materials, including polymers and resins.
- Employed in the preparation of catalysts for various organic transformations.
Biology:
- Investigated for its potential use in bio-compatible materials and drug delivery systems.
Medicine:
- Explored for its applications in the development of novel pharmaceuticals and diagnostic agents.
Industry:
- Utilized in the production of high-performance coatings, adhesives, and sealants.
- Applied in the manufacture of electronic components and devices.
Mechanism of Action
The mechanism of action of bis(pentafluorophenyl)diethoxysilane is primarily based on its ability to act as a Lewis acid. The silicon atom, being electron-deficient, can coordinate with electron-rich species, facilitating various chemical transformations. The pentafluorophenyl groups enhance the electron-withdrawing nature of the silicon atom, increasing its reactivity.
Molecular Targets and Pathways:
Lewis Acid Catalysis: Activation of substrates through coordination with the silicon atom.
Cross-Coupling Reactions: Formation of carbon-silicon bonds via palladium-catalyzed mechanisms.
Comparison with Similar Compounds
Bis(pentafluorophenyl)borane: Similar in structure but contains boron instead of silicon.
Tris(pentafluorophenyl)borane: Contains three pentafluorophenyl groups attached to boron.
Pentafluorophenylsilane: Contains a single pentafluorophenyl group attached to silicon.
Uniqueness:
- Dimethoxybis(pentafluorophenyl)silane is unique due to the presence of two pentafluorophenyl groups and two ethoxy groups, providing a balance of reactivity and stability.
- The compound’s ability to undergo a variety of chemical reactions makes it versatile for different applications in research and industry.
Properties
Molecular Formula |
C16H10F10O2Si |
---|---|
Molecular Weight |
452.32 g/mol |
IUPAC Name |
diethoxy-bis(2,3,4,5,6-pentafluorophenyl)silane |
InChI |
InChI=1S/C16H10F10O2Si/c1-3-27-29(28-4-2,15-11(23)7(19)5(17)8(20)12(15)24)16-13(25)9(21)6(18)10(22)14(16)26/h3-4H2,1-2H3 |
InChI Key |
XJYMDGOGWVHLFW-UHFFFAOYSA-N |
Canonical SMILES |
CCO[Si](C1=C(C(=C(C(=C1F)F)F)F)F)(C2=C(C(=C(C(=C2F)F)F)F)F)OCC |
Origin of Product |
United States |
Synthesis routes and methods I
Procedure details
Synthesis routes and methods II
Procedure details
Disclaimer and Information on In-Vitro Research Products
Please be aware that all articles and product information presented on BenchChem are intended solely for informational purposes. The products available for purchase on BenchChem are specifically designed for in-vitro studies, which are conducted outside of living organisms. In-vitro studies, derived from the Latin term "in glass," involve experiments performed in controlled laboratory settings using cells or tissues. It is important to note that these products are not categorized as medicines or drugs, and they have not received approval from the FDA for the prevention, treatment, or cure of any medical condition, ailment, or disease. We must emphasize that any form of bodily introduction of these products into humans or animals is strictly prohibited by law. It is essential to adhere to these guidelines to ensure compliance with legal and ethical standards in research and experimentation.