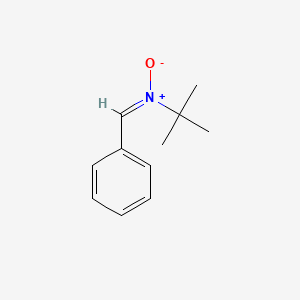
Phenyl-N-tert-butylnitrone, (E)-
Overview
Description
Phenyl-N-tert-butylnitrone (PBN), a spin-trapping compound with the chemical structure (E)-N-tert-butyl-α-phenylnitrone, is a potent antioxidant and neuroprotective agent. It functions by trapping reactive oxygen species (ROS) and free radicals, forming stable adducts that mitigate oxidative stress, a key contributor to neurodegenerative diseases, ischemia-reperfusion injury, and retinal degeneration . PBN’s efficacy extends to inhibiting pro-inflammatory pathways (e.g., NF-κB and COX-2) and enhancing endogenous antioxidant systems . Its ability to cross the blood-brain barrier (BBB) and protect neuronal cells in preclinical models has established it as a benchmark for nitrone-based therapeutics .
Preparation Methods
Synthetic Routes and Reaction Conditions: Phenyl-N-tert-butylnitrone, (E)-, can be synthesized through the condensation of N-tert-butylhydroxylamine with benzaldehyde. The reaction typically involves the use of an acid catalyst and proceeds under mild conditions . The general reaction scheme is as follows:
N-tert-butylhydroxylamine+benzaldehyde→Phenyl-N-tert-butylnitrone, (E)-
Industrial Production Methods: In industrial settings, the synthesis of Phenyl-N-tert-butylnitrone, (E)-, follows similar principles but is scaled up to accommodate larger production volumes. The reaction conditions are optimized to ensure high yield and purity of the final product .
Chemical Reactions Analysis
Types of Reactions: Phenyl-N-tert-butylnitrone, (E)-, undergoes various chemical reactions, including:
Oxidation: The compound can be oxidized to form corresponding oxides.
Reduction: It can be reduced to amines under specific conditions.
Substitution: The nitrone group can participate in nucleophilic substitution reactions.
Common Reagents and Conditions:
Oxidation: Common oxidizing agents include hydrogen peroxide and potassium permanganate.
Reduction: Reducing agents such as lithium aluminum hydride are often used.
Substitution: Nucleophiles like amines and thiols can react with the nitrone group.
Major Products Formed:
Oxidation: Formation of oxides.
Reduction: Formation of amines.
Substitution: Formation of substituted nitrones.
Scientific Research Applications
Pharmacological Properties
PBN has been extensively studied for its pharmacological effects across various conditions. Key findings include:
- Protection Against Oxidative Stress : PBN acts as a free radical scavenger, which helps mitigate oxidative damage in various biological systems. It has shown protective effects against ischemia-reperfusion injury, endotoxin shock, and neurodegenerative diseases .
- Neuroprotection : Research indicates that PBN can protect neuronal cells from oxidative stress and apoptosis in models of stroke and neurodegenerative diseases. For instance, it has been shown to prevent light-induced neurodegeneration in retinal cells by inhibiting the enzyme RPE65, which is crucial for the visual cycle .
- Anti-inflammatory Effects : PBN exhibits anti-inflammatory properties by inhibiting lipoxygenase activity, which is significant in managing inflammatory diseases .
Neuroprotective Applications
PBN's neuroprotective properties have made it a focus of research for treating various neurological conditions:
- Stroke : PBN has been investigated for its ability to reduce infarct size and improve recovery outcomes in animal models of stroke. Studies suggest that it prevents secondary energy failure and mitochondrial dysfunction following ischemic events .
- Neurodegenerative Diseases : The compound has shown promise in models of Alzheimer's disease and Parkinson's disease by reducing oxidative stress and improving neuronal survival. Its antioxidant capacity plays a critical role in these protective effects .
Case Studies and Experimental Findings
Numerous studies have documented the efficacy of PBN in various experimental settings:
- Retinal Protection : A study demonstrated that administering PBN prior to light exposure significantly protected rat retinas from damage, suggesting its potential application in age-related macular degeneration .
- Chemotherapy-Induced Neuropathic Pain : Research indicated that PBN could alleviate neuropathic pain induced by chemotherapy agents, highlighting its role as a supportive therapy in cancer treatment .
- Stroke Models : In vivo studies have shown that PBN administration before ischemic events leads to improved outcomes, including reduced cell death and enhanced functional recovery post-stroke .
Synthesis and Mechanism of Action
PBN is synthesized through specific chemical reactions involving nitrones. Its mechanism primarily involves trapping reactive oxygen species (ROS), thus preventing cellular damage caused by oxidative stress. The compound's ability to react with these radicals results in the formation of less reactive species, contributing to its protective effects against cellular injury .
Comparative Efficacy of Derivatives
Recent studies have explored derivatives of PBN to enhance its therapeutic potential:
Compound | Activity | IC50 (µM) | Notes |
---|---|---|---|
PBN | Antioxidant | 0.1 | Standard reference compound |
HBN1 | Neuroprotection | 87.57 | Effective in oxygen-glucose deprivation models |
HBN2 | Antinecrotic | 3.47 | Exhibits strong anti-apoptotic properties |
These derivatives demonstrate varying degrees of efficacy, suggesting that modifications to the PBN structure can lead to improved pharmacological profiles.
Mechanism of Action
The primary mechanism of action of Phenyl-N-tert-butylnitrone, (E)-, involves its ability to trap free radicals. The nitrone group reacts with free radicals to form stable spin adducts, which can be detected and analyzed using electron spin resonance spectroscopy . Additionally, the compound has been shown to inhibit the activity of certain enzymes, such as cyclooxygenase-2, thereby exerting anti-inflammatory effects .
Comparison with Similar Compounds
Comparison with Structural Analogues
Homo-Bis-Nitrones (HBNs) and Homo-Tris-Nitrones (HTNs)
HTNs, derived from PBN by adding multiple nitrone groups, exhibit variable neuroprotective efficacy. In SH-SY5Y neuroblastoma models under oligomycin A/rotenone (O/R) stress, HTN2 (N-tert-butyl-substituted) showed the lowest EC50 (8.2 µM) and maximal neuroprotection (92%), outperforming PBN (EC50: 14.5 µM; 85% activity). However, HTN1 (N-methyl) and HTN3 (N-benzyl) displayed reduced potency, suggesting substituent bulkiness inversely affects efficacy .
Key Findings :
- Antiapoptotic Activity : HTN3 demonstrated superior inhibition of caspase-3 (30% reduction vs. PBN’s 20%) and LDH release (40% vs. 35%), linked to its N-benzyl group enhancing radical scavenging .
Biphenylnitrones (BPNs)
BPNs, featuring a biphenyl scaffold, showed improved radical scavenging over PBN in oxygen-glucose deprivation (OGD) models. BPN5 exhibited an EC50 of 5.8 µM (vs. PBN’s 14.5 µM) and 95% maximal neuroprotection, attributed to enhanced lipophilicity and electron-donating groups .
Comparison with Non-Nitrone Antioxidants
N-Acetylcysteine (NAC)
NAC, a glutathione precursor, showed comparable maximal neuroprotection (85%) to PBN but required higher doses (EC50: 25 µM). Unlike PBN, NAC lacks direct spin-trapping capability, relying on indirect ROS neutralization .
Nordihydroguaiaretic Acid (NDGA)
NDGA, a lipoxygenase inhibitor, demonstrated stronger anti-inflammatory effects (70% LOX inhibition vs. PBN’s 50%) but inferior BBB penetration, limiting its utility in cerebral ischemia .
Pharmacokinetic and Clinical Comparisons
Cerovive (NXY-059)
Cerovive, a sulfonated PBN derivative, failed Phase III trials due to poor BBB penetration and rapid renal clearance. Despite superior in vitro ROS scavenging (EC50: 6.7 µM), its pharmacokinetic limitations underscored PBN’s relative advantages in bioavailability .
Lipophilic Derivatives (4-F-PBN, 4-CF3-PBN)
Derivatives like 4-F-PBN exhibited 3-fold greater RPE65 inhibition (IC50: 2.1 µM vs. PBN’s 6.5 µM), slowing visual cycle progression and protecting against light-induced retinal damage. Enhanced lipophilicity improved retinal accumulation .
Mechanisms of Action Comparison
Compound | Key Mechanisms | ROS Scavenging Efficiency (EC50, µM) | BBB Penetration |
---|---|---|---|
PBN | Spin-trapping, NF-κB/COX-2 inhibition, endogenous antioxidant induction | 14.5 | High |
HTN3 | Enhanced antiapoptotic activity via N-benzyl group | 12.8 | Moderate |
BPN5 | Biphenyl structure enhances electron delocalization | 5.8 | High |
NAC | Glutathione synthesis, indirect ROS neutralization | 25.0 | Low |
Cerovive | Sulfonate groups improve solubility but reduce BBB access | 6.7 | Low |
Data Tables
Table 1: Neuroprotective Efficacy in OGD Models
Compound | EC50 (µM) | Maximal Activity (%) | Caspase-3 Inhibition (%) |
---|---|---|---|
PBN | 14.5 | 85 | 20 |
HTN2 | 8.2 | 92 | 25 |
HTN3 | 12.8 | 90 | 30 |
BPN5 | 5.8 | 95 | 35 |
NAC | 25.0 | 85 | 15 |
Table 2: Retinal Protection Efficacy
Compound | RPE65 Inhibition (IC50, µM) | Photoreceptor Survival (%) |
---|---|---|
PBN | 6.5 | 80 |
4-F-PBN | 2.1 | 92 |
4-CF3-PBN | 1.8 | 95 |
Biological Activity
Phenyl-N-tert-butylnitrone (PBN) is a nitrone compound that has garnered attention due to its potential neuroprotective and antioxidant properties. This article explores the biological activity of PBN, focusing on its mechanisms of action, effects in various biological models, and its derivatives.
PBN functions primarily as a free radical trap , which allows it to mitigate oxidative stress. Its ability to scavenge reactive oxygen species (ROS) plays a crucial role in its neuroprotective effects. Research indicates that PBN can effectively reduce superoxide production in neuronal cultures, thereby protecting against oxidative damage .
Neuroprotective Effects
PBN has been evaluated in various experimental models for its neuroprotective capabilities:
- In Vitro Studies : PBN demonstrated significant neuroprotection against oxidative stress induced by glutamate toxicity in rat cerebellar neurons, with an effective concentration (EC50) of 2.7 mM . Additionally, it reduced cell death in human neuroblastoma SH-SY5Y cells exposed to metabolic inhibitors, with EC50 values reported as low as 11.2 μM .
- In Vivo Studies : In animal models of ischemic stroke, PBN administration resulted in reduced infarct size and improved neurological outcomes. For instance, in a transient middle cerebral artery occlusion (MCAO) model, PBN significantly decreased the volume of cerebral infarction when administered at various time points post-ischemia .
Antioxidant Activity
The antioxidant properties of PBN are well-documented. It has been shown to scavenge hydroxyl radicals and inhibit lipid peroxidation effectively. Comparative studies with its derivatives indicate that certain modifications enhance its antioxidant capacity. For example, 4-hydroxyphenyl-N-tert-butyl nitrone exhibited superior protective effects against hepatocarcinogenesis compared to PBN itself .
Derivatives and Structure-Activity Relationships
Research has also focused on derivatives of PBN to evaluate their biological activities:
- 4-Hydroxyphenyl-N-tert-butyl nitrone : This derivative was found to selectively induce apoptosis in preneoplastic hepatocyte populations, highlighting its potential for cancer prevention .
- Homo-tris-nitrones : These compounds derived from PBN showed varying degrees of neuroprotective potency, with some exhibiting better efficacy than PBN at specific concentrations .
Table 1: Comparative Biological Activity of PBN and Its Derivatives
Compound | EC50 (μM) | Neuroprotective Activity | Antioxidant Activity |
---|---|---|---|
Phenyl-N-tert-butylnitrone (PBN) | 11.2 | High | Moderate |
4-Hydroxyphenyl-N-tert-butyl nitrone | 9.5 | Very High | High |
Homo-tris-nitrone HTN2 | 10.0 | Moderate | High |
Homo-tris-nitrone HTN3 | 8.0 | Very High | Very High |
Case Studies
- Stroke Models : In studies involving MCAO, rats treated with PBN showed a significant reduction in infarct size compared to controls. The percentage of infarct volume was reduced from 22.7% in untreated groups to approximately 9.6% in treated groups .
- Hepatocarcinogenesis : A study on Wistar rats indicated that dietary inclusion of phenyl-N-tert-butylnitrone significantly decreased the size and number of preneoplastic lesions under conditions promoting liver cancer development .
Q & A
Basic Research Questions
Q. What are the established synthetic routes for (E)-phenyl-N-tert-butylnitrone (PBN), and how can purity be optimized?
PBN is typically synthesized via condensation reactions between benzaldehyde derivatives and tert-butylamine, followed by oxidation. Key steps include:
- Condensation : Benzaldehyde reacts with tert-butylamine in ethanol under reflux, forming an imine intermediate .
- Oxidation : The imine is oxidized using hydrogen peroxide or m-chloroperbenzoic acid (mCPBA) to yield the nitrone .
- Purification : Recrystallization from hexane/ethyl acetate mixtures or silica gel chromatography ensures high purity (>95%) .
Critical Parameters : Reaction temperature (60–80°C) and stoichiometric control of the oxidizing agent are essential to minimize byproducts like N-oxide dimers .
Q. How is PBN utilized as a spin trap in electron spin resonance (ESR) spectroscopy for radical detection?
PBN reacts with transient free radicals (e.g., hydroxyl, lipid-derived radicals) to form stable spin adducts detectable via ESR. Methodological considerations include:
- Radical Trapping : Use 10–50 mM PBN in aqueous or lipid-rich media, depending on radical solubility .
- ESR Parameters : Typical settings: microwave power 10–20 mW, modulation amplitude 1 G, and scan range 100 G. Adducts exhibit hyperfine splitting constants (aN ≈ 14–16 G, aH ≈ 2–3 G) .
- Validation : Confirm specificity using radical scavengers (e.g., ascorbate for superoxide) and control experiments without irradiation/oxidants .
Q. What experimental models are suitable for evaluating PBN’s neuroprotective effects?
- In Vivo Ischemia Models : Transient middle cerebral artery occlusion (tMCAO) in rodents, with PBN administered intraperitoneally (50–100 mg/kg) pre- or post-injury. Endpoints include infarct volume (TTC staining) and mitochondrial function assays .
- Oxidative Stress Assays : Measure lipid peroxidation (MDA levels via TBARS) and glutathione (GSH/GSSG ratios) in brain homogenates .
Advanced Research Questions
Q. How can structural modifications of PBN enhance its therapeutic efficacy in mitochondrial-targeted antioxidant therapy?
Amphiphilic PBN derivatives improve mitochondrial membrane penetration. Key strategies:
- Hydrophilic-Lipophilic Balance (HLB) : Attach polar groups (e.g., lactobionamide) to the nitrone core while retaining tert-butyl hydrophobicity. Derivatives with logP values of 1.5–2.5 show optimal mitochondrial uptake .
- In Vitro Validation : Use rotenone-induced ATPase deficiency models in fibroblasts. Measure ATP synthesis (luciferase assays) and ROS levels (DCFH-DA fluorescence) .
Q. How do researchers reconcile contradictory findings on PBN’s efficacy in retinal degeneration models?
PBN protects against light-induced retinal damage but fails to rescue genetic degeneration (e.g., P23H rhodopsin mutations). Methodological insights:
- Model Selection : Light damage models involve acute ROS bursts, while genetic models (e.g., S334ter rats) involve chronic protein misfolding. Use ERG and immunohistochemistry to assess photoreceptor survival .
- Mechanistic Divergence : PBN scavenges ROS but does not correct mutant rhodopsin trafficking. Combine with gene therapy (e.g., AAV-mediated rhodopsin delivery) for synergistic effects .
Q. What advanced techniques validate PBN’s role in inhibiting NLRP3 inflammasome activation?
- Microglial Cultures : Treat BV-2 cells with LPS/ATP to activate NLRP3. Pre-incubate with PBN (10–50 µM) and measure IL-1β release (ELISA) and caspase-1 activity (Western blot) .
- In Vivo Hyperalgesia Models : Sleep-deprived rodents administered PBN (30 mg/kg, i.p.) show reduced spinal NLRP3 expression (qPCR) and microglial activation (Iba1 staining) .
Q. How does PBN mitigate teratogenicity in developmental toxicity studies?
In thalidomide-induced embryopathy models (e.g., rabbit embryos), PBN (40 mg/kg, maternal injection) reduces limb malformations by 60%. Mechanistic assays:
- DNA Oxidative Damage : Quantify 8-OHdG levels in embryonic tissues via LC-MS .
- Redox Profiling : Measure SOD/CAT activity and GSH levels to confirm ROS suppression .
Q. What are non-conventional radical generation methods for studying PBN adducts?
- Photolytic Methods : Irradiate chlorotricyanomethane/PBN mixtures at −30°C to generate tricyanomethyl-PBN adducts. Use low temperatures to prevent cycloaddition side reactions .
- Sensitized Photo-oxidation : Employ 2,4,6-tris(4-methoxyphenyl)pyrylium ion as a photosensitizer for controlled radical generation in organic solvents .
Q. Methodological Considerations for Data Interpretation
Q. How should researchers address variability in PBN’s pharmacokinetics across studies?
- Dose Optimization : Conduct bioavailability studies using LC-MS to measure plasma/brain PBN levels. Typical t1/2 ≈ 2–4 hours in rodents .
- Species Differences : Adjust dosing intervals in non-rodent models (e.g., rabbits) based on metabolic rate .
Q. What criteria distinguish artifact signals from genuine spin adducts in ESR experiments?
Properties
IUPAC Name |
N-tert-butyl-1-phenylmethanimine oxide | |
---|---|---|
Details | Computed by Lexichem TK 2.7.0 (PubChem release 2021.05.07) | |
Source | PubChem | |
URL | https://pubchem.ncbi.nlm.nih.gov | |
Description | Data deposited in or computed by PubChem | |
InChI |
InChI=1S/C11H15NO/c1-11(2,3)12(13)9-10-7-5-4-6-8-10/h4-9H,1-3H3/b12-9+ | |
Details | Computed by InChI 1.0.6 (PubChem release 2021.05.07) | |
Source | PubChem | |
URL | https://pubchem.ncbi.nlm.nih.gov | |
Description | Data deposited in or computed by PubChem | |
InChI Key |
IYSYLWYGCWTJSG-FMIVXFBMSA-N | |
Details | Computed by InChI 1.0.6 (PubChem release 2021.05.07) | |
Source | PubChem | |
URL | https://pubchem.ncbi.nlm.nih.gov | |
Description | Data deposited in or computed by PubChem | |
Canonical SMILES |
CC(C)(C)[N+](=CC1=CC=CC=C1)[O-] | |
Details | Computed by OEChem 2.3.0 (PubChem release 2021.05.07) | |
Source | PubChem | |
URL | https://pubchem.ncbi.nlm.nih.gov | |
Description | Data deposited in or computed by PubChem | |
Isomeric SMILES |
CC(C)(C)/[N+](=C\C1=CC=CC=C1)/[O-] | |
Details | Computed by OEChem 2.3.0 (PubChem release 2021.05.07) | |
Source | PubChem | |
URL | https://pubchem.ncbi.nlm.nih.gov | |
Description | Data deposited in or computed by PubChem | |
Molecular Formula |
C11H15NO | |
Details | Computed by PubChem 2.1 (PubChem release 2021.05.07) | |
Source | PubChem | |
URL | https://pubchem.ncbi.nlm.nih.gov | |
Description | Data deposited in or computed by PubChem | |
Molecular Weight |
177.24 g/mol | |
Details | Computed by PubChem 2.1 (PubChem release 2021.05.07) | |
Source | PubChem | |
URL | https://pubchem.ncbi.nlm.nih.gov | |
Description | Data deposited in or computed by PubChem | |
CAS No. |
85225-53-2, 3376-24-7 | |
Record name | Phenyl-N-tert-butylnitrone, (E)- | |
Source | ChemIDplus | |
URL | https://pubchem.ncbi.nlm.nih.gov/substance/?source=chemidplus&sourceid=0085225532 | |
Description | ChemIDplus is a free, web search system that provides access to the structure and nomenclature authority files used for the identification of chemical substances cited in National Library of Medicine (NLM) databases, including the TOXNET system. | |
Record name | 2-Propanamine, 2-methyl-N-(phenylmethylene)-, N-oxide | |
Source | EPA Chemicals under the TSCA | |
URL | https://www.epa.gov/chemicals-under-tsca | |
Description | EPA Chemicals under the Toxic Substances Control Act (TSCA) collection contains information on chemicals and their regulations under TSCA, including non-confidential content from the TSCA Chemical Substance Inventory and Chemical Data Reporting. | |
Record name | PHENYL-N-TERT-BUTYLNITRONE, (E)- | |
Source | FDA Global Substance Registration System (GSRS) | |
URL | https://gsrs.ncats.nih.gov/ginas/app/beta/substances/UEW4MZ1486 | |
Description | The FDA Global Substance Registration System (GSRS) enables the efficient and accurate exchange of information on what substances are in regulated products. Instead of relying on names, which vary across regulatory domains, countries, and regions, the GSRS knowledge base makes it possible for substances to be defined by standardized, scientific descriptions. | |
Explanation | Unless otherwise noted, the contents of the FDA website (www.fda.gov), both text and graphics, are not copyrighted. They are in the public domain and may be republished, reprinted and otherwise used freely by anyone without the need to obtain permission from FDA. Credit to the U.S. Food and Drug Administration as the source is appreciated but not required. | |
Synthesis routes and methods I
Procedure details
Synthesis routes and methods II
Procedure details
Synthesis routes and methods III
Procedure details
Synthesis routes and methods IV
Procedure details
Retrosynthesis Analysis
AI-Powered Synthesis Planning: Our tool employs the Template_relevance Pistachio, Template_relevance Bkms_metabolic, Template_relevance Pistachio_ringbreaker, Template_relevance Reaxys, Template_relevance Reaxys_biocatalysis model, leveraging a vast database of chemical reactions to predict feasible synthetic routes.
One-Step Synthesis Focus: Specifically designed for one-step synthesis, it provides concise and direct routes for your target compounds, streamlining the synthesis process.
Accurate Predictions: Utilizing the extensive PISTACHIO, BKMS_METABOLIC, PISTACHIO_RINGBREAKER, REAXYS, REAXYS_BIOCATALYSIS database, our tool offers high-accuracy predictions, reflecting the latest in chemical research and data.
Strategy Settings
Precursor scoring | Relevance Heuristic |
---|---|
Min. plausibility | 0.01 |
Model | Template_relevance |
Template Set | Pistachio/Bkms_metabolic/Pistachio_ringbreaker/Reaxys/Reaxys_biocatalysis |
Top-N result to add to graph | 6 |
Feasible Synthetic Routes
Disclaimer and Information on In-Vitro Research Products
Please be aware that all articles and product information presented on BenchChem are intended solely for informational purposes. The products available for purchase on BenchChem are specifically designed for in-vitro studies, which are conducted outside of living organisms. In-vitro studies, derived from the Latin term "in glass," involve experiments performed in controlled laboratory settings using cells or tissues. It is important to note that these products are not categorized as medicines or drugs, and they have not received approval from the FDA for the prevention, treatment, or cure of any medical condition, ailment, or disease. We must emphasize that any form of bodily introduction of these products into humans or animals is strictly prohibited by law. It is essential to adhere to these guidelines to ensure compliance with legal and ethical standards in research and experimentation.