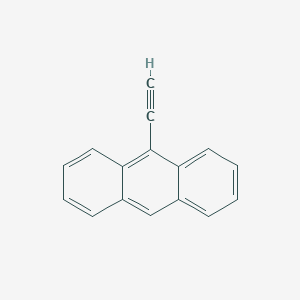
9-Ethynylanthracene
Overview
Description
9-Ethynylanthracene, also known as this compound, is a useful research compound. Its molecular formula is C16H10 and its molecular weight is 202.25 g/mol. The purity is usually 95%.
BenchChem offers high-quality this compound suitable for many research applications. Different packaging options are available to accommodate customers' requirements. Please inquire for more information about this compound including the price, delivery time, and more detailed information at info@benchchem.com.
Safety and Hazards
Future Directions
Mechanism of Action
Target of Action
9-Ethynylanthracene is a chemical compound with the molecular formula C16H10
Mode of Action
It’s important to note that the interaction of a compound with its targets can lead to various biochemical changes, which can have significant effects on cellular functions .
Biochemical Pathways
This compound has been used in the synthesis of di-ynes using Eglinton’s oxidative coupling reaction . This suggests that it may interact with biochemical pathways involved in oxidative processes.
Result of Action
Understanding these effects requires detailed studies on the compound’s interaction with its targets and the resulting biochemical changes .
Action Environment
The action of this compound may be influenced by various environmental factors. For instance, it has been suggested that the compound should be stored in an inert atmosphere at 2-8°C . This implies that factors such as temperature and atmospheric composition could potentially influence the compound’s action, efficacy, and stability .
Properties
IUPAC Name |
9-ethynylanthracene | |
---|---|---|
Source | PubChem | |
URL | https://pubchem.ncbi.nlm.nih.gov | |
Description | Data deposited in or computed by PubChem | |
InChI |
InChI=1S/C16H10/c1-2-14-15-9-5-3-7-12(15)11-13-8-4-6-10-16(13)14/h1,3-11H | |
Source | PubChem | |
URL | https://pubchem.ncbi.nlm.nih.gov | |
Description | Data deposited in or computed by PubChem | |
InChI Key |
WSZBYXQREMPYLP-UHFFFAOYSA-N | |
Source | PubChem | |
URL | https://pubchem.ncbi.nlm.nih.gov | |
Description | Data deposited in or computed by PubChem | |
Canonical SMILES |
C#CC1=C2C=CC=CC2=CC3=CC=CC=C31 | |
Source | PubChem | |
URL | https://pubchem.ncbi.nlm.nih.gov | |
Description | Data deposited in or computed by PubChem | |
Molecular Formula |
C16H10 | |
Source | PubChem | |
URL | https://pubchem.ncbi.nlm.nih.gov | |
Description | Data deposited in or computed by PubChem | |
DSSTOX Substance ID |
DTXSID10399811 | |
Record name | 9-ethynylanthracene | |
Source | EPA DSSTox | |
URL | https://comptox.epa.gov/dashboard/DTXSID10399811 | |
Description | DSSTox provides a high quality public chemistry resource for supporting improved predictive toxicology. | |
Molecular Weight |
202.25 g/mol | |
Source | PubChem | |
URL | https://pubchem.ncbi.nlm.nih.gov | |
Description | Data deposited in or computed by PubChem | |
CAS No. |
13752-40-4 | |
Record name | 9-ethynylanthracene | |
Source | EPA DSSTox | |
URL | https://comptox.epa.gov/dashboard/DTXSID10399811 | |
Description | DSSTox provides a high quality public chemistry resource for supporting improved predictive toxicology. | |
Synthesis routes and methods I
Procedure details
Synthesis routes and methods II
Procedure details
Q1: What are some of the synthetic routes available for producing 9-Ethynylanthracene?
A1: this compound can be synthesized through various methods. One common approach involves converting 9-acetylanthracene to 9,10-dichloroanthracene using phosphorus pentachloride, followed by a reaction sequence based on the Kobrich method to yield the desired this compound [].
Q2: What is the typical polymerization behavior of this compound?
A2: this compound can be polymerized using various initiators, such as complex phosphinic, arsinic, and stibinic initiators like NiCl2′2PPh3 [, ]. Polymerization typically occurs at elevated temperatures, around 80°C. The resulting product often consists of benzene-soluble and benzene-insoluble fractions. The benzene-insoluble fraction tends to exhibit a conjugated polyenic structure. In contrast, the soluble fraction, with a lower molecular weight, often incorporates dihydroanthracene units within the polymer backbone alongside conjugated double bonds [].
Q3: How does the choice of initiator influence the stereochemistry of the resulting poly(this compound)?
A3: The use of phosphinic, arsinic, and stibinic initiators generally favors the formation of cis isomers in poly(this compound) [].
Q4: What thermal transitions are observed in poly(this compound)?
A4: Thermal analysis techniques like DTA and DSC reveal a cis to trans isomerization in poly(this compound) occurring within the temperature range of 260-290°C [].
Q5: Can this compound be used as a building block for more complex structures?
A5: Yes, this compound serves as a versatile precursor in organic synthesis. For instance, it can be employed in Eglinton's oxidative coupling reaction to generate corresponding di-ynes []. Furthermore, derivatives like 2,9-Diethynylanthracene can be synthesized and further reacted to produce compounds like 2,9-bis(phenylethynyl)anthracenes and dianthrylbutadiynes [].
Q6: How does the structure of this compound influence its NMR spectrum?
A6: The presence of the ethynyl group in this compound significantly affects its proton NMR spectrum. Analysis of the proton chemical shifts, in comparison to other acetylenes, reveals contributions from the magnetic anisotropy and steric effects of the acetylene group, in addition to electronic effects impacting nearby protons [].
Q7: Can this compound be incorporated into organometallic complexes?
A7: Absolutely, this compound acts as a ligand in organometallic chemistry. For instance, it can react with platinum(II) terpyridyl complexes to form mononuclear platinum complexes like [(tpy)Pt−C≡C–C14H9]PF6 (where C14H9 represents the 9-anthryl group) [].
Q8: How does this compound contribute to materials with aggregation-induced emission properties?
A8: this compound plays a crucial role in modulating the luminescence behavior of organogold(I) complexes. When introduced as a ligand alongside AIE-active ligands, this compound can influence the complex's luminescence, potentially leading to aggregation-caused quenching (ACQ) []. This highlights its potential in designing materials with tunable emission properties.
Q9: Has this compound been utilized in studying rotational isomerism?
A9: Yes, this compound has been incorporated into molecules like bis(1,4-disubstituted 9-triptycyl)ethynes to study rotational isomerism around C(sp)-C(sp3) bonds []. These studies provide valuable insights into the factors affecting rotational barriers in molecules containing acetylenic linkages.
Q10: Does this compound have applications in supramolecular chemistry?
A10: Research indicates the potential of this compound in supramolecular chemistry. For instance, it can be employed as a component in synthetic replicators capable of driving propagating reaction-diffusion fronts []. This highlights its potential in developing self-replicating systems and exploring complex chemical phenomena.
Q11: What is the historical context of this compound research?
A11: Research on this compound and related compounds has been ongoing for several decades. Early work explored the synthesis and polymerization of this compound, investigating its potential as a precursor for photoconductive polymers []. Over time, the scope of research expanded to encompass various aspects, including its use in organometallic chemistry, supramolecular chemistry, and materials science.
Disclaimer and Information on In-Vitro Research Products
Please be aware that all articles and product information presented on BenchChem are intended solely for informational purposes. The products available for purchase on BenchChem are specifically designed for in-vitro studies, which are conducted outside of living organisms. In-vitro studies, derived from the Latin term "in glass," involve experiments performed in controlled laboratory settings using cells or tissues. It is important to note that these products are not categorized as medicines or drugs, and they have not received approval from the FDA for the prevention, treatment, or cure of any medical condition, ailment, or disease. We must emphasize that any form of bodily introduction of these products into humans or animals is strictly prohibited by law. It is essential to adhere to these guidelines to ensure compliance with legal and ethical standards in research and experimentation.