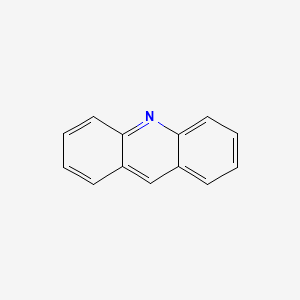
Acridine
Overview
Description
Acridine is an organic compound and a nitrogen heterocycle with the formula C₁₃H₉N. It is a planar molecule structurally related to anthracene, with one of the central CH groups replaced by nitrogen. This compound is mildly basic and is almost colorless, crystallizing in needles. Historically, this compound was first isolated from coal tar by Carl Gräbe and Heinrich Caro in 1870 . It has a distinctive acrid odor and skin-irritating properties.
Synthetic Routes and Reaction Conditions:
Ullmann Condensation Reaction: One common method involves the Ullmann condensation reaction of 2-bromobenzoic acid with different anilines to produce 2-arylamino benzoic acids.
Industrial Production Methods:
Coal Tar Extraction: this compound is separated from coal tar by extracting with dilute sulfuric acid.
Types of Reactions:
Oxidation: this compound can undergo oxidation reactions, forming acridone.
Reduction: It can be reduced to dihydrothis compound.
Substitution: this compound can participate in substitution reactions, particularly at the nitrogen atom.
Common Reagents and Conditions:
Oxidation: Potassium permanganate or chromium trioxide can be used as oxidizing agents.
Reduction: Sodium borohydride or lithium aluminum hydride are common reducing agents.
Substitution: Halogenation can be achieved using halogens like chlorine or bromine in the presence of a catalyst.
Major Products:
Oxidation: Acridone.
Reduction: Dihydrothis compound.
Substitution: Halogenated acridines.
Scientific Research Applications
Acridine and its derivatives have a broad range of applications in various fields:
Chemistry: Used as intermediates in organic synthesis and as dyes.
Biology: this compound derivatives are used as fluorescent probes for nucleic acids.
Industry: Historically used as pigments and dyes, and more recently as corrosion inhibitors.
Mechanism of Action
Acridine is often compared with other nitrogen-containing heterocycles such as:
Quinoline: Similar in structure but with different biological activities.
Phenanthridine: Another nitrogen heterocycle with distinct properties.
Acridone: A direct oxidation product of this compound with unique applications.
Uniqueness: this compound’s unique planar structure and ability to intercalate with DNA make it particularly valuable in medicinal chemistry for developing anticancer agents .
Comparison with Similar Compounds
- Quinoline
- Phenanthridine
- Acridone
Acridine’s versatility and wide range of applications make it a compound of significant interest in both scientific research and industrial applications.
Properties
CAS No. |
39327-16-7 |
---|---|
Molecular Formula |
C13H9N |
Molecular Weight |
179.22 g/mol |
IUPAC Name |
benzo[g]quinoline |
InChI |
InChI=1S/C13H9N/c1-2-5-11-9-13-12(6-3-7-14-13)8-10(11)4-1/h1-9H |
InChI Key |
RFQDDXWZZVRLKO-UHFFFAOYSA-N |
SMILES |
C1=CC=C2C(=C1)C=C3C=CC=CC3=N2 |
Canonical SMILES |
C1=CC=C2C=C3C(=CC2=C1)C=CC=N3 |
boiling_point |
655 °F at 760 mmHg (NTP, 1992) 345.5 °C @ 760 mm Hg 655 °F |
Color/Form |
RHOMBOHEDRAL NEEDLES OR PRISMS FROM ALCOHOL; MONOCLINIC, ORTHORHOMBIC SMALL COLORLESS NEEDLES ORTHORHOMBIC PLATES, NEEDLES FROM DILUTED ALCOHOL SMALL, COLORLESS OR FAINTLY YELLOW CRYSTALS |
density |
1.2 at 68 °F (approx.) (USCG, 1999) - Denser than water; will sink 1.005 @ 20 °C/4 °C 1.2 |
melting_point |
225 to 230 °F (NTP, 1992) 111 °C; 106 °C (form a); 110 °C (form b) 225-230 °F |
physical_description |
Small colorless needle-like crystalline solid. Slightly soluble in hot water. Slightly denser than water. Contact may irritate skin, eyes, and mucous membranes. Sublimes before melting when heated. May be toxic by ingestion. Pale yellow solid; [Merck Index] Colorless solid; [Hawley] Yellow crystalline powder; [MSDSonline] Small colorless needle-like crystalline solid. |
solubility |
SLIGHTLY SOL IN HOT WATER; VERY SOL IN ALCOHOL, ETHER, BENZENE AND CARBON DISULFIDE Freely sol in hydrocarbons; slightly soluble in boiling water, liquid ammonia, liguid sulfur dioxide; sparingly soluble in light petroleum Very soluble in carbon disulfide; very soluble in ethanol, ether, benzene; slightly soluble in water 1 gm soluble in <1 ml boiling benzene or alcohol; 5 ml benzene (20 °C); 6 ml alcohol (20 °C); 16 ml of ether (20 °C); 1.8 ml boiling cyclohexane. In water= 38.4 mg/L at 24 °C. |
vapor_pressure |
1 mmHg at 255.9 °F (NTP, 1992) Vapor pressure= 1.35X10-4 mm Hg at 25 °C /extrapolated from experimentally-derived coefficients/ 1 MM HG @ 129 °C varies depending upon the specific compound |
Origin of Product |
United States |
Synthesis routes and methods I
Procedure details
Synthesis routes and methods II
Procedure details
Synthesis routes and methods III
Procedure details
Synthesis routes and methods IV
Procedure details
Synthesis routes and methods V
Procedure details
Retrosynthesis Analysis
AI-Powered Synthesis Planning: Our tool employs the Template_relevance Pistachio, Template_relevance Bkms_metabolic, Template_relevance Pistachio_ringbreaker, Template_relevance Reaxys, Template_relevance Reaxys_biocatalysis model, leveraging a vast database of chemical reactions to predict feasible synthetic routes.
One-Step Synthesis Focus: Specifically designed for one-step synthesis, it provides concise and direct routes for your target compounds, streamlining the synthesis process.
Accurate Predictions: Utilizing the extensive PISTACHIO, BKMS_METABOLIC, PISTACHIO_RINGBREAKER, REAXYS, REAXYS_BIOCATALYSIS database, our tool offers high-accuracy predictions, reflecting the latest in chemical research and data.
Strategy Settings
Precursor scoring | Relevance Heuristic |
---|---|
Min. plausibility | 0.01 |
Model | Template_relevance |
Template Set | Pistachio/Bkms_metabolic/Pistachio_ringbreaker/Reaxys/Reaxys_biocatalysis |
Top-N result to add to graph | 6 |
Feasible Synthetic Routes
Disclaimer and Information on In-Vitro Research Products
Please be aware that all articles and product information presented on BenchChem are intended solely for informational purposes. The products available for purchase on BenchChem are specifically designed for in-vitro studies, which are conducted outside of living organisms. In-vitro studies, derived from the Latin term "in glass," involve experiments performed in controlled laboratory settings using cells or tissues. It is important to note that these products are not categorized as medicines or drugs, and they have not received approval from the FDA for the prevention, treatment, or cure of any medical condition, ailment, or disease. We must emphasize that any form of bodily introduction of these products into humans or animals is strictly prohibited by law. It is essential to adhere to these guidelines to ensure compliance with legal and ethical standards in research and experimentation.