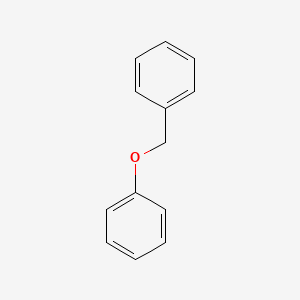
Benzyl phenyl ether
Overview
Description
Benzyl phenyl ether is an organic compound with the molecular formula C13H12O. It is a type of ether, characterized by an oxygen atom connected to two aryl groups, specifically a benzyl group and a phenyl group. This compound is commonly used as a model compound in catalytic chemistry to represent the alpha-O4 ether bond in lignin and coal .
Synthetic Routes and Reaction Conditions:
Williamson Ether Synthesis: This is the most common method for preparing ethers, including this compound. It involves the reaction of an alkoxide ion with a primary alkyl halide or tosylate in an S_N2 reaction.
Industrial Production Methods: Industrially, ethers like this compound can be synthesized through the acid-catalyzed dehydration of alcohols.
Types of Reactions:
Oxidation: this compound can undergo oxidation reactions, often resulting in the formation of phenol and benzaldehyde.
Reduction: The compound can be reduced to form benzyl alcohol and phenol.
Common Reagents and Conditions:
Oxidation: Common oxidizing agents include potassium permanganate (KMnO4) and chromium trioxide (CrO3).
Reduction: Reducing agents such as lithium aluminum hydride (LiAlH4) and sodium borohydride (NaBH4) are typically used.
Substitution: Aluminum bromide (AlBr3) in chlorobenzene is a common reagent for substitution reactions involving this compound.
Major Products:
Oxidation: Phenol and benzaldehyde.
Reduction: Benzyl alcohol and phenol.
Substitution: Phenol, ortho-benzyl phenol, and dichlorodiphenylmethane.
Mechanism of Action
Target of Action
Benzyl phenyl ether is a reactive organic oxygenate, containing an ether as a functional group . It is present in subbituminous and bituminous coals . The primary targets of this compound are the ether bonds in lignin and coal .
Mode of Action
The most common reaction of ethers like this compound is the cleavage of the C–O bond by using strong acids . During acidic cleavage, the ether oxygen is protonated to form a good leaving group, which can be eliminated as part of an SN2, SN1, or E1 reaction mechanism . The mechanistic pathway is primarily determined by the strong acid used and the type of substituents attached to the ether .
Biochemical Pathways
The biochemical pathway of this compound involves the cleavage of the ether bond, forming phenol and benzyl alcohol as intermediates, followed by C-C bond alkylation . This reaction is catalyzed by hydronium ions, which are provided by the dissociation of water at high temperatures .
Pharmacokinetics
It’s known that the compound’s reactivity and subsequent transformations are influenced by the presence of strong acids .
Result of Action
The result of the action of this compound is the cleavage of the ether bond, leading to the formation of phenol and benzyl alcohol . This reaction represents the a-O4 ether bond in lignin and coal .
Action Environment
The action of this compound is influenced by environmental factors such as temperature and the presence of strong acids . For instance, the cleavage of the C-O bond in this compound occurs at high temperatures . Additionally, the presence of strong acids like HI or HBr facilitates the cleavage of the ether bond .
Biochemical Analysis
Biochemical Properties
Benzyl phenyl ether plays a significant role in biochemical reactions, particularly in the hydrogenolysis of lignin, a major component of woody biomass . The hydrogenolysis reaction network closely follows that of solution-based reactions, with the primary products being toluene, phenol, and cyclohexanol .
Molecular Mechanism
The molecular mechanism of this compound involves its hydrogenolysis over supported nickel catalysts at room temperature and atmospheric hydrogen pressure . The mechanical energy during milling not only drives the chemical reactions but also activates the nickel by exposing fresh metallic surfaces from passivated particles .
Temporal Effects in Laboratory Settings
In laboratory settings, the effects of this compound over time are primarily observed in its role in the hydrogenolysis of lignin. The hydrogenolysis rate is shown to be largely insensitive to the final nickel particle size .
Metabolic Pathways
This compound is involved in the metabolic pathways of lignin deconstruction, contributing to the production of toluene, phenol, and cyclohexanol .
Scientific Research Applications
Catalytic Applications
Hydrogenolysis of Benzyl Phenyl Ether
One of the primary applications of BPE is in catalytic hydrogenolysis, particularly as a model compound for studying lignin degradation. In recent studies, BPE has been subjected to mechanocatalytic hydrogenolysis using nickel catalysts. This method allows for solvent-free reactions that mimic the cleavage of C–O bonds found in lignin .
Mechanism and Products
The hydrogenolysis of BPE typically yields products such as:
- Toluene
- Phenol
- Cyclohexanol
These products are generated through the cleavage of the benzyl ether bond, demonstrating BPE's utility in biomass valorization and the development of sustainable chemical processes .
Experimental Insights
Research indicates that the reaction pathways for BPE closely resemble those observed in thermally catalyzed reactions. The mechanical energy from ball milling enhances the catalytic activity by exposing fresh metallic surfaces on nickel catalysts, thereby facilitating the reaction at room temperature .
Analytical Applications
Nuclear Magnetic Resonance (NMR) Spectroscopy
This compound is also utilized in NMR spectroscopy as a reference compound due to its well-defined spectral characteristics. Advanced techniques such as HSQC (Heteronuclear Single Quantum Coherence) NMR provide insights into complex mixtures involving BPE, allowing for enhanced resolution and quantification of metabolites .
Quantitative Analysis
The application of quantitative NMR techniques has been pivotal in metabolomics, enabling researchers to analyze the concentration of various compounds in biological samples effectively. BPE serves as a model compound for developing these analytical methods, which can be applied across various fields including food science and clinical diagnostics .
Material Science Applications
Polymer Chemistry
This compound has been investigated for its role in polymer chemistry, particularly in the synthesis of polymeric materials through etherification reactions. Its reactivity allows for the modification of polymer properties, making it valuable in creating specialty polymers with tailored functionalities .
Reactivity with Other Compounds
Research has shown that BPE can undergo various reactions such as:
- Photo-Claisen rearrangements : This process involves the rearrangement of BPE under UV light in the presence of zeolites, leading to new polymeric structures with unique properties .
- Decomposition Reactions : Studies have explored the decomposition pathways of BPE under superheated conditions, providing insights into its behavior in high-temperature processes relevant to materials science and energy applications .
Pharmaceutical Applications
Drug Development
This compound derivatives have been explored for their potential pharmaceutical applications. Research has indicated that modifications to the BPE structure can lead to compounds with biological activity, highlighting its importance as a scaffold in drug design .
Case Studies
Several studies have documented the synthesis and evaluation of this compound derivatives for their pharmacological properties, demonstrating their potential use as therapeutic agents against various diseases .
Comparison with Similar Compounds
Anisole (methyl phenyl ether): Anisole is similar to benzyl phenyl ether but has a methoxy group instead of a benzyl group.
Phenyl ethyl ether: This compound has an ethyl group instead of a benzyl group.
Diphenyl ether: This compound has two phenyl groups attached to the oxygen atom.
Uniqueness: this compound is unique due to its specific structure, which includes both a benzyl and a phenyl group. This structure makes it particularly useful as a model compound for studying the alpha-O4 ether bond in lignin and coal, which is not as effectively represented by other similar compounds .
Biological Activity
Benzyl phenyl ether (BPE) is an organic compound with significant implications in various biological and chemical processes. This article explores the biological activity of BPE, focusing on its mechanisms, effects, and potential applications in different fields.
This compound is characterized by its ether functional group, which contributes to its reactivity and interaction with biological systems. Its molecular structure allows it to participate in various chemical reactions, including hydrolysis and hydrogenolysis, which can lead to the formation of biologically active metabolites.
-
Hydrolysis and Hydrogenolysis :
- In aqueous environments, BPE undergoes hydrolysis, leading to the formation of phenol and benzyl alcohol. This reaction is catalyzed by hydronium ions and can be significantly accelerated in the presence of catalysts such as Ni/SiO2 or HZSM-5 .
- The cleavage of the ether bond has been demonstrated to be a critical pathway for the biological activity of BPE, affecting its pharmacological properties.
-
Antifouling Activity :
- Recent studies have shown that certain derivatives of phenyl ether, including BPE, exhibit potent anti-larval settlement activity against barnacles (e.g., Balanus amphitrite). The effective concentration (EC50) values for these compounds were reported to be lower than 3.05 μM, indicating their potential as environmentally friendly antifouling agents .
Study 1: Blood Pressure Effects
A study examined the effects of related compounds derived from BPE on blood pressure in male cats. The parent compound exhibited significant blood pressure-lowering effects at a dose of 1 mg/kg, suggesting potential therapeutic applications in cardiovascular health .
Study 2: Solvolysis Reactions
Research on the solvolysis of BPE in high-temperature aqueous ethanol solutions revealed that this process can yield monoaromatic compounds such as benzyl alcohol and phenol. The highest yield achieved was 76.7% under optimized conditions (598 K and high-pressure CO2), highlighting BPE's utility in producing valuable chemical intermediates .
Data Table: Biological Activities of this compound Derivatives
Compound | Activity | EC50 (μM) | Notes |
---|---|---|---|
This compound | Antifouling | < 3.05 | Effective against barnacle settlement |
3'-hydroxy-4'-(beta-diethylaminoethoxy)-3',4'-dihydroseselin | Blood Pressure Lowering | 1 mg/kg | Significant effects observed in feline models |
Polybrominated diphenyl ether derivative | Antifouling | Not specified | Considered a promising antifouling agent |
Properties
IUPAC Name |
phenoxymethylbenzene | |
---|---|---|
Source | PubChem | |
URL | https://pubchem.ncbi.nlm.nih.gov | |
Description | Data deposited in or computed by PubChem | |
InChI |
InChI=1S/C13H12O/c1-3-7-12(8-4-1)11-14-13-9-5-2-6-10-13/h1-10H,11H2 | |
Source | PubChem | |
URL | https://pubchem.ncbi.nlm.nih.gov | |
Description | Data deposited in or computed by PubChem | |
InChI Key |
BOTNYLSAWDQNEX-UHFFFAOYSA-N | |
Source | PubChem | |
URL | https://pubchem.ncbi.nlm.nih.gov | |
Description | Data deposited in or computed by PubChem | |
Canonical SMILES |
C1=CC=C(C=C1)COC2=CC=CC=C2 | |
Source | PubChem | |
URL | https://pubchem.ncbi.nlm.nih.gov | |
Description | Data deposited in or computed by PubChem | |
Molecular Formula |
C13H12O | |
Source | PubChem | |
URL | https://pubchem.ncbi.nlm.nih.gov | |
Description | Data deposited in or computed by PubChem | |
DSSTOX Substance ID |
DTXSID70870813 | |
Record name | Benzyloxybenzene | |
Source | EPA DSSTox | |
URL | https://comptox.epa.gov/dashboard/DTXSID70870813 | |
Description | DSSTox provides a high quality public chemistry resource for supporting improved predictive toxicology. | |
Molecular Weight |
184.23 g/mol | |
Source | PubChem | |
URL | https://pubchem.ncbi.nlm.nih.gov | |
Description | Data deposited in or computed by PubChem | |
CAS No. |
946-80-5, 31324-44-4 | |
Record name | Benzyl phenyl ether | |
Source | ChemIDplus | |
URL | https://pubchem.ncbi.nlm.nih.gov/substance/?source=chemidplus&sourceid=0000946805 | |
Description | ChemIDplus is a free, web search system that provides access to the structure and nomenclature authority files used for the identification of chemical substances cited in National Library of Medicine (NLM) databases, including the TOXNET system. | |
Record name | Phenoxytoluene | |
Source | ChemIDplus | |
URL | https://pubchem.ncbi.nlm.nih.gov/substance/?source=chemidplus&sourceid=0031324444 | |
Description | ChemIDplus is a free, web search system that provides access to the structure and nomenclature authority files used for the identification of chemical substances cited in National Library of Medicine (NLM) databases, including the TOXNET system. | |
Record name | Benzyl phenyl ether | |
Source | DTP/NCI | |
URL | https://dtp.cancer.gov/dtpstandard/servlet/dwindex?searchtype=NSC&outputformat=html&searchlist=77971 | |
Description | The NCI Development Therapeutics Program (DTP) provides services and resources to the academic and private-sector research communities worldwide to facilitate the discovery and development of new cancer therapeutic agents. | |
Explanation | Unless otherwise indicated, all text within NCI products is free of copyright and may be reused without our permission. Credit the National Cancer Institute as the source. | |
Record name | Benzyloxybenzene | |
Source | EPA DSSTox | |
URL | https://comptox.epa.gov/dashboard/DTXSID70870813 | |
Description | DSSTox provides a high quality public chemistry resource for supporting improved predictive toxicology. | |
Record name | Benzyl Phenyl Ether | |
Source | European Chemicals Agency (ECHA) | |
URL | https://echa.europa.eu/information-on-chemicals | |
Description | The European Chemicals Agency (ECHA) is an agency of the European Union which is the driving force among regulatory authorities in implementing the EU's groundbreaking chemicals legislation for the benefit of human health and the environment as well as for innovation and competitiveness. | |
Explanation | Use of the information, documents and data from the ECHA website is subject to the terms and conditions of this Legal Notice, and subject to other binding limitations provided for under applicable law, the information, documents and data made available on the ECHA website may be reproduced, distributed and/or used, totally or in part, for non-commercial purposes provided that ECHA is acknowledged as the source: "Source: European Chemicals Agency, http://echa.europa.eu/". Such acknowledgement must be included in each copy of the material. ECHA permits and encourages organisations and individuals to create links to the ECHA website under the following cumulative conditions: Links can only be made to webpages that provide a link to the Legal Notice page. | |
Record name | BENZYL PHENYL ETHER | |
Source | FDA Global Substance Registration System (GSRS) | |
URL | https://gsrs.ncats.nih.gov/ginas/app/beta/substances/BUE863N0L8 | |
Description | The FDA Global Substance Registration System (GSRS) enables the efficient and accurate exchange of information on what substances are in regulated products. Instead of relying on names, which vary across regulatory domains, countries, and regions, the GSRS knowledge base makes it possible for substances to be defined by standardized, scientific descriptions. | |
Explanation | Unless otherwise noted, the contents of the FDA website (www.fda.gov), both text and graphics, are not copyrighted. They are in the public domain and may be republished, reprinted and otherwise used freely by anyone without the need to obtain permission from FDA. Credit to the U.S. Food and Drug Administration as the source is appreciated but not required. | |
Synthesis routes and methods I
Procedure details
Synthesis routes and methods II
Procedure details
Synthesis routes and methods III
Procedure details
Synthesis routes and methods IV
Procedure details
Synthesis routes and methods V
Procedure details
Retrosynthesis Analysis
AI-Powered Synthesis Planning: Our tool employs the Template_relevance Pistachio, Template_relevance Bkms_metabolic, Template_relevance Pistachio_ringbreaker, Template_relevance Reaxys, Template_relevance Reaxys_biocatalysis model, leveraging a vast database of chemical reactions to predict feasible synthetic routes.
One-Step Synthesis Focus: Specifically designed for one-step synthesis, it provides concise and direct routes for your target compounds, streamlining the synthesis process.
Accurate Predictions: Utilizing the extensive PISTACHIO, BKMS_METABOLIC, PISTACHIO_RINGBREAKER, REAXYS, REAXYS_BIOCATALYSIS database, our tool offers high-accuracy predictions, reflecting the latest in chemical research and data.
Strategy Settings
Precursor scoring | Relevance Heuristic |
---|---|
Min. plausibility | 0.01 |
Model | Template_relevance |
Template Set | Pistachio/Bkms_metabolic/Pistachio_ringbreaker/Reaxys/Reaxys_biocatalysis |
Top-N result to add to graph | 6 |
Feasible Synthetic Routes
Q1: What is the molecular formula and weight of benzyl phenyl ether?
A1: this compound has the molecular formula C13H12O and a molecular weight of 184.23 g/mol.
Q2: Does spectroscopic data exist for this compound?
A: Yes, various spectroscopic techniques have been employed to characterize BPE, including infrared (IR) spectroscopy, which confirms the presence of the characteristic ether (C–O) bond. []
Q3: Is this compound stable at high temperatures?
A: BPE undergoes thermal decomposition at elevated temperatures. Studies have investigated its pyrolysis behavior in various environments, including supercritical fluids like water and methanol. [] The stability of BPE is influenced by factors like temperature, solvent, and the presence of catalysts.
Q4: How does confinement affect the pyrolysis of this compound?
A: Research indicates that confining BPE within the nanopores of mesoporous silica materials like MCM-41 can alter its pyrolysis pathways and product distribution compared to pyrolysis on nonporous silica. [] This confinement effect is attributed to the restricted diffusion and altered interactions of reactive species within the confined space.
Q5: Can this compound be used to study lignin depolymerization?
A: Yes, BPE serves as a model compound to investigate the catalytic cleavage of aryl ether linkages, which are prevalent in lignin. Understanding BPE reactivity provides insights into strategies for breaking down lignin into valuable aromatic compounds. [, , ]
Q6: What catalysts are effective for this compound hydrogenolysis?
A: Various catalysts have been explored for BPE hydrogenolysis, including those based on palladium, nickel, and copper. The choice of catalyst influences the reaction rate, selectivity, and reaction conditions required. [, , ]
Q7: How does the addition of samarium(III) or formic acid affect the reactivity of copper-doped porous metal oxides towards this compound?
A: Research has shown that adding samarium(III) directly into the porous metal oxide structure enhances the hydrogenolysis of aryl ether linkages in BPE. Conversely, adding soluble samarium(III) salts suppresses this activity. Formic acid, on the other hand, appears to deactivate basic sites on the catalyst, which hinders hydrogenolysis but improves selectivity toward aromatic products by mitigating undesirable hydrogenation reactions. []
Q8: Can this compound conversion be used to produce high carbon number hydrocarbon fuels?
A: Research indicates that BPE can be converted into larger phenolic compounds (C12-C22) through a one-step process involving C–O bond breaking and C–C coupling, catalyzed by metal triflates. [] These larger phenolic compounds hold potential as precursors for phenol-formaldehyde polymers, diesel/jet fuels, and liquid organic hydrogen carriers.
Q9: Have computational methods been applied to study this compound reactions?
A: Yes, density functional theory (DFT) calculations have been employed to investigate the mechanisms of BPE reactions, such as C–O bond cleavage. [] These calculations provide insights into the electronic factors governing reactivity and can aid in catalyst design.
Q10: How does modifying the structure of this compound affect its reactivity?
A: Studies have explored the influence of substituents on the aromatic rings of BPE on its reactivity in reactions like C–O bond cleavage. [] Electronic effects of the substituents can influence the electron density distribution in the molecule, impacting its interaction with catalysts and, consequently, its reactivity.
Q11: Are there strategies to enhance the stability of this compound?
A11: While BPE itself might not be inherently unstable, its reactivity under certain conditions, like high temperatures, necessitates careful consideration. The choice of solvent and the presence or absence of catalysts significantly impact its stability during reactions.
Q12: Are there specific SHE regulations concerning this compound?
A12: While BPE itself might not be subject to specific regulations like those for pharmaceuticals, standard laboratory safety protocols apply due to its potential flammability and the possibility of producing harmful byproducts during reactions. Researchers should consult relevant safety data sheets and follow appropriate laboratory safety practices.
Q13: What analytical techniques are used to study this compound reactions?
A: Gas chromatography (GC) and gas chromatography-mass spectrometry (GC-MS) are commonly employed to analyze the products of BPE reactions, allowing for the identification and quantification of different compounds formed. [] Operando magic-angle spinning NMR spectroscopy provides real-time insights into the reaction process, offering valuable kinetic and mechanistic information. []
Q14: Are there alternative model compounds for studying lignin reactivity?
A: Yes, other model compounds like 2-phenylethyl phenyl ether, diphenyl ether, biphenyl, and 2,3-dihydrobenzofuran are used to mimic different types of linkages found in lignin, allowing researchers to study a broader range of chemical transformations relevant to lignin valorization. [, ]
Disclaimer and Information on In-Vitro Research Products
Please be aware that all articles and product information presented on BenchChem are intended solely for informational purposes. The products available for purchase on BenchChem are specifically designed for in-vitro studies, which are conducted outside of living organisms. In-vitro studies, derived from the Latin term "in glass," involve experiments performed in controlled laboratory settings using cells or tissues. It is important to note that these products are not categorized as medicines or drugs, and they have not received approval from the FDA for the prevention, treatment, or cure of any medical condition, ailment, or disease. We must emphasize that any form of bodily introduction of these products into humans or animals is strictly prohibited by law. It is essential to adhere to these guidelines to ensure compliance with legal and ethical standards in research and experimentation.