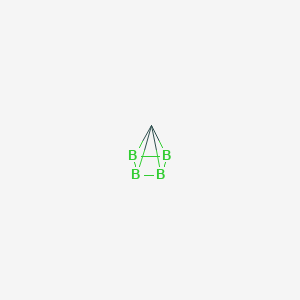
Boron carbide
Overview
Description
Boron carbide is a crystalline compound of boron and carbon, with the chemical formula B₄C. It is an extremely hard material, ranking third after diamond and cubic boron nitride in terms of hardness. This compound is known for its low density, high melting point, and exceptional chemical stability. It is widely used in various industrial applications, including abrasives, wear-resistant materials, and control rods for nuclear reactors .
Scientific Research Applications
Boron carbide has a wide range of applications in scientific research:
Chemistry: Used as a catalyst in various chemical reactions due to its high surface area and chemical stability.
Biology: Investigated for its potential use in boron neutron capture therapy (BNCT) for cancer treatment.
Industry: Widely used in the production of wear-resistant materials, abrasives, and lightweight armor.
Mechanism of Action
Target of Action
Boron carbide (B4C) is a crystalline compound of boron and carbon . It is an extremely hard, synthetically produced material that is used in various applications. The primary targets of this compound are materials that require abrasion resistance, such as tank armor, bulletproof vests, and engine sabotage powders . It is also used in control rods for nuclear power generation due to its neutron-absorbing properties .
Mode of Action
This compound interacts with its targets primarily through its hardness and resistance to wear. It is one of the hardest known materials, exceeded only by cubic boron nitride and diamond . This extreme hardness allows it to provide superior protection and durability when used in applications such as armor and wear-resistant products . In nuclear reactors, this compound acts as a neutron absorber, controlling the rate of fission .
Biochemical Pathways
Research has suggested that this compound nanoparticles can be used as carriers for boron delivery to the tumor microenvironment in boron neutron capture therapy . This involves the use of macrophages, which show a greater ability to interact with this compound than other cell types .
Pharmacokinetics
The pharmacokinetics of this compound, particularly in the context of boron neutron capture therapy, is an area of ongoing research. Macrophages loaded with this compound nanoparticles have been shown to migrate efficiently, suggesting potential for targeted delivery of therapeutic agents . .
Result of Action
The primary result of this compound’s action is the provision of hardness and wear resistance. When used in armor and similar applications, it provides effective protection against damage . In the context of nuclear power generation, this compound helps control the rate of fission, contributing to the safe and effective operation of nuclear reactors .
Safety and Hazards
Boron carbide is considered hazardous by the 2012 OSHA Hazard Communication Standard . It may cause acute inhalation toxicity - dusts and mists, skin corrosion/irritation, serious eye damage/eye irritation, and specific target organ toxicity (single exposure) with the target organs being the respiratory system .
Future Directions
The global boron carbide market size was valued at USD 347.21 million in 2020 and is estimated to exhibit a CAGR of 4.9% from 2021 to 2027 . Increasing construction of nuclear reactors for sustainable energy in emerging countries is expected to drive the product demand during the forecast timeframe .
Preparation Methods
Synthetic Routes and Reaction Conditions: Boron carbide can be synthesized through several methods:
Carbothermal Reduction: This is the primary industrial method, involving the reduction of boron oxide (B₂O₃) with carbon at high temperatures (above 2000°C). The reaction is as follows: [ 2B₂O₃ + 7C \rightarrow B₄C + 6CO ]
Chemical Vapor Deposition (CVD): This method involves the reaction of boron halides (such as boron trichloride) with methane or other carbon sources in the presence of hydrogen at temperatures between 400°C and 600°C. .
Self-Propagating High-Temperature Synthesis (SHS): This method involves the exothermic reaction of boron and carbon powders, which propagates through the reactant mixture, producing this compound.
Industrial Production Methods: The carbothermal reduction method is the most widely used for industrial production due to its cost-effectiveness and scalability. The process involves heating a mixture of boron oxide and carbon in an electric furnace at temperatures exceeding 2000°C. The resulting this compound is then ground into a fine powder and can be further processed into various forms, such as sintered ceramics or composite materials .
Types of Reactions:
Oxidation: this compound can undergo oxidation at high temperatures, forming boron oxide and carbon dioxide. The reaction is as follows: [ 2B₄C + 7O₂ \rightarrow 2B₂O₃ + 4CO₂ ]
Reduction: this compound can be reduced by metals such as magnesium, producing elemental boron and magnesium carbide.
Substitution: this compound can react with other carbides or nitrides, forming mixed compounds with unique properties.
Common Reagents and Conditions:
Oxidation: Typically occurs at temperatures above 600°C in the presence of oxygen.
Reduction: Requires a reducing agent such as magnesium and high temperatures (above 1000°C).
Substitution: Involves reacting this compound with other carbides or nitrides at high temperatures (above 1500°C).
Major Products:
Oxidation: Produces boron oxide and carbon dioxide.
Reduction: Produces elemental boron and magnesium carbide.
Substitution: Produces mixed boron-carbide-nitride compounds
Comparison with Similar Compounds
Boron carbide is unique among boron compounds due to its combination of hardness, low density, and high chemical stability. Similar compounds include:
Cubic Boron Nitride (cBN): Similar in hardness to this compound but has a higher thermal stability and is used in cutting tools and abrasives.
Hexagonal Boron Nitride (hBN): Known for its lubricating properties and high thermal conductivity, used in high-temperature lubricants and thermal management applications.
Boron Nitride Nanotubes (BNNTs): Similar to carbon nanotubes but with higher thermal stability and chemical resistance, used in advanced composite materials and nanotechnology applications
This compound stands out due to its unique combination of properties, making it a versatile material for a wide range of applications.
Properties
IUPAC Name |
2,3,4,5-tetraboratetracyclo[2.1.0.01,3.02,5]pentane | |
---|---|---|
Source | PubChem | |
URL | https://pubchem.ncbi.nlm.nih.gov | |
Description | Data deposited in or computed by PubChem | |
InChI |
InChI=1S/CB4/c2-1-3(2)5(1)4(1)2 | |
Source | PubChem | |
URL | https://pubchem.ncbi.nlm.nih.gov | |
Description | Data deposited in or computed by PubChem | |
InChI Key |
INAHAJYZKVIDIZ-UHFFFAOYSA-N | |
Source | PubChem | |
URL | https://pubchem.ncbi.nlm.nih.gov | |
Description | Data deposited in or computed by PubChem | |
Canonical SMILES |
B12B3C14B2B43 | |
Source | PubChem | |
URL | https://pubchem.ncbi.nlm.nih.gov | |
Description | Data deposited in or computed by PubChem | |
Molecular Formula |
B4C, CB4 | |
Record name | boron carbide | |
Source | Wikipedia | |
URL | https://en.wikipedia.org/wiki/Boron_carbide | |
Description | Chemical information link to Wikipedia. | |
Source | PubChem | |
URL | https://pubchem.ncbi.nlm.nih.gov | |
Description | Data deposited in or computed by PubChem | |
DSSTOX Substance ID |
DTXSID4051615 | |
Record name | Boron carbide | |
Source | EPA DSSTox | |
URL | https://comptox.epa.gov/dashboard/DTXSID4051615 | |
Description | DSSTox provides a high quality public chemistry resource for supporting improved predictive toxicology. | |
Molecular Weight |
55.3 g/mol | |
Source | PubChem | |
URL | https://pubchem.ncbi.nlm.nih.gov | |
Description | Data deposited in or computed by PubChem | |
Physical Description |
Dry Powder, Black shiny solid; [Merck Index] Black odorless powder; Insoluble in water; [Alfa Aesar MSDS] | |
Record name | Boron carbide (B4C) | |
Source | EPA Chemicals under the TSCA | |
URL | https://www.epa.gov/chemicals-under-tsca | |
Description | EPA Chemicals under the Toxic Substances Control Act (TSCA) collection contains information on chemicals and their regulations under TSCA, including non-confidential content from the TSCA Chemical Substance Inventory and Chemical Data Reporting. | |
Record name | Boron carbide | |
Source | Haz-Map, Information on Hazardous Chemicals and Occupational Diseases | |
URL | https://haz-map.com/Agents/9702 | |
Description | Haz-Map® is an occupational health database designed for health and safety professionals and for consumers seeking information about the adverse effects of workplace exposures to chemical and biological agents. | |
Explanation | Copyright (c) 2022 Haz-Map(R). All rights reserved. Unless otherwise indicated, all materials from Haz-Map are copyrighted by Haz-Map(R). No part of these materials, either text or image may be used for any purpose other than for personal use. Therefore, reproduction, modification, storage in a retrieval system or retransmission, in any form or by any means, electronic, mechanical or otherwise, for reasons other than personal use, is strictly prohibited without prior written permission. | |
CAS No. |
12069-32-8, 12316-49-3, 60063-34-5 | |
Record name | Boron carbide | |
Source | ChemIDplus | |
URL | https://pubchem.ncbi.nlm.nih.gov/substance/?source=chemidplus&sourceid=0012069328 | |
Description | ChemIDplus is a free, web search system that provides access to the structure and nomenclature authority files used for the identification of chemical substances cited in National Library of Medicine (NLM) databases, including the TOXNET system. | |
Record name | Tetra(10B)boron carbide | |
Source | ChemIDplus | |
URL | https://pubchem.ncbi.nlm.nih.gov/substance/?source=chemidplus&sourceid=0012316493 | |
Description | ChemIDplus is a free, web search system that provides access to the structure and nomenclature authority files used for the identification of chemical substances cited in National Library of Medicine (NLM) databases, including the TOXNET system. | |
Record name | Boron carbide (B4C) | |
Source | EPA Chemicals under the TSCA | |
URL | https://www.epa.gov/chemicals-under-tsca | |
Description | EPA Chemicals under the Toxic Substances Control Act (TSCA) collection contains information on chemicals and their regulations under TSCA, including non-confidential content from the TSCA Chemical Substance Inventory and Chemical Data Reporting. | |
Record name | Boron carbide | |
Source | EPA DSSTox | |
URL | https://comptox.epa.gov/dashboard/DTXSID4051615 | |
Description | DSSTox provides a high quality public chemistry resource for supporting improved predictive toxicology. | |
Record name | Boron carbide | |
Source | European Chemicals Agency (ECHA) | |
URL | https://echa.europa.eu/substance-information/-/substanceinfo/100.031.907 | |
Description | The European Chemicals Agency (ECHA) is an agency of the European Union which is the driving force among regulatory authorities in implementing the EU's groundbreaking chemicals legislation for the benefit of human health and the environment as well as for innovation and competitiveness. | |
Explanation | Use of the information, documents and data from the ECHA website is subject to the terms and conditions of this Legal Notice, and subject to other binding limitations provided for under applicable law, the information, documents and data made available on the ECHA website may be reproduced, distributed and/or used, totally or in part, for non-commercial purposes provided that ECHA is acknowledged as the source: "Source: European Chemicals Agency, http://echa.europa.eu/". Such acknowledgement must be included in each copy of the material. ECHA permits and encourages organisations and individuals to create links to the ECHA website under the following cumulative conditions: Links can only be made to webpages that provide a link to the Legal Notice page. | |
Record name | Boron carbide | |
Source | European Chemicals Agency (ECHA) | |
URL | https://echa.europa.eu/substance-information/-/substanceinfo/100.056.383 | |
Description | The European Chemicals Agency (ECHA) is an agency of the European Union which is the driving force among regulatory authorities in implementing the EU's groundbreaking chemicals legislation for the benefit of human health and the environment as well as for innovation and competitiveness. | |
Explanation | Use of the information, documents and data from the ECHA website is subject to the terms and conditions of this Legal Notice, and subject to other binding limitations provided for under applicable law, the information, documents and data made available on the ECHA website may be reproduced, distributed and/or used, totally or in part, for non-commercial purposes provided that ECHA is acknowledged as the source: "Source: European Chemicals Agency, http://echa.europa.eu/". Such acknowledgement must be included in each copy of the material. ECHA permits and encourages organisations and individuals to create links to the ECHA website under the following cumulative conditions: Links can only be made to webpages that provide a link to the Legal Notice page. | |
Record name | Tetra[10B]boron carbide | |
Source | European Chemicals Agency (ECHA) | |
URL | https://echa.europa.eu/substance-information/-/substanceinfo/100.032.335 | |
Description | The European Chemicals Agency (ECHA) is an agency of the European Union which is the driving force among regulatory authorities in implementing the EU's groundbreaking chemicals legislation for the benefit of human health and the environment as well as for innovation and competitiveness. | |
Explanation | Use of the information, documents and data from the ECHA website is subject to the terms and conditions of this Legal Notice, and subject to other binding limitations provided for under applicable law, the information, documents and data made available on the ECHA website may be reproduced, distributed and/or used, totally or in part, for non-commercial purposes provided that ECHA is acknowledged as the source: "Source: European Chemicals Agency, http://echa.europa.eu/". Such acknowledgement must be included in each copy of the material. ECHA permits and encourages organisations and individuals to create links to the ECHA website under the following cumulative conditions: Links can only be made to webpages that provide a link to the Legal Notice page. | |
Record name | BORON CARBIDE | |
Source | FDA Global Substance Registration System (GSRS) | |
URL | https://gsrs.ncats.nih.gov/ginas/app/beta/substances/T5V24LJ508 | |
Description | The FDA Global Substance Registration System (GSRS) enables the efficient and accurate exchange of information on what substances are in regulated products. Instead of relying on names, which vary across regulatory domains, countries, and regions, the GSRS knowledge base makes it possible for substances to be defined by standardized, scientific descriptions. | |
Explanation | Unless otherwise noted, the contents of the FDA website (www.fda.gov), both text and graphics, are not copyrighted. They are in the public domain and may be republished, reprinted and otherwise used freely by anyone without the need to obtain permission from FDA. Credit to the U.S. Food and Drug Administration as the source is appreciated but not required. | |
Disclaimer and Information on In-Vitro Research Products
Please be aware that all articles and product information presented on BenchChem are intended solely for informational purposes. The products available for purchase on BenchChem are specifically designed for in-vitro studies, which are conducted outside of living organisms. In-vitro studies, derived from the Latin term "in glass," involve experiments performed in controlled laboratory settings using cells or tissues. It is important to note that these products are not categorized as medicines or drugs, and they have not received approval from the FDA for the prevention, treatment, or cure of any medical condition, ailment, or disease. We must emphasize that any form of bodily introduction of these products into humans or animals is strictly prohibited by law. It is essential to adhere to these guidelines to ensure compliance with legal and ethical standards in research and experimentation.