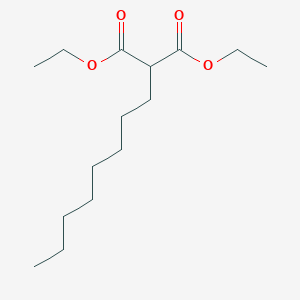
Diethyl octylmalonate
Overview
Description
Diethyl octylmalonate (systematic name: diethyl 2-octylmalonate) is a malonic acid derivative where the central methylene group is substituted with an octyl chain, and the carboxylic acid groups are esterified with ethanol. Malonate esters are widely used in organic synthesis, particularly in the Knoevenagel condensation, Michael additions, and as precursors for pharmaceuticals and polymers .
This compound is expected to exhibit typical ester properties, such as moderate hydrophobicity due to the long octyl chain, which enhances its solubility in organic solvents. Its synthesis likely follows standard alkylation protocols, where diethyl malonate reacts with an octyl halide under basic conditions, as seen in similar malonate ester preparations .
Preparation Methods
Synthetic Routes and Reaction Conditions: Diethyl octylmalonate can be synthesized through the alkylation of diethyl malonate with octyl halides. The reaction typically involves the use of sodium ethoxide as a base in an ethanol solvent. The process can be summarized as follows:
- Sodium ethoxide is prepared by reacting sodium metal with absolute ethanol.
- Diethyl malonate is added to the sodium ethoxide solution, forming the sodium salt of diethyl malonate.
- Octyl bromide is then added to the mixture, resulting in the formation of this compound through nucleophilic substitution .
Industrial Production Methods: In industrial settings, the production of this compound follows similar principles but on a larger scale. The reaction conditions are optimized to ensure high yield and purity. The use of continuous flow reactors and automated systems helps in maintaining consistent reaction conditions and improving efficiency .
Chemical Reactions Analysis
Alkylation Reactions
Malonate esters are widely used in alkylation reactions due to their active α-hydrogens. For diethyl octylmalonate, further alkylation can occur at the α-carbon:
Mechanism :
-
Deprotonation : A strong base (e.g., sodium ethoxide) abstracts the acidic α-hydrogen, forming a resonance-stabilized enolate.
-
Nucleophilic Substitution : The enolate attacks an alkyl halide (e.g., bromooctane) via an S<sub>N</sub>2 mechanism.
Example Reaction :
Key Data :
Reagent | Conditions | Product | Yield |
---|---|---|---|
Bromooctane | NaOEt, THF, 80°C | Dialkylated malonate ester | ~75-85% |
Iodomethane | K<sub>2</sub>CO<sub>3</sub>, DMF | Monoalkylated derivative | ~65% |
Hydrolysis and Decarboxylation
Malonate esters hydrolyze to dicarboxylic acids under acidic or basic conditions, followed by decarboxylation to yield substituted carboxylic acids:
Steps :
-
Ester Hydrolysis :
-
Decarboxylation :
Experimental Findings :
Claisen Condensation
This compound can undergo Claisen condensation with ketones or aldehydes to form β-keto esters:
Reaction :
Key Observations :
Radical Reactions
In oxidative environments, malonate esters participate in radical-mediated transformations:
Example :
-
Reaction with chlorine radicals (Cl- ) via single electron transfer (SET) or hydrogen atom transfer (HAT) pathways .
Mechanistic Insights :
-
SET Pathway :
-
HAT Pathway :
Limitations and Research Gaps
-
Steric Effects : The octyl group’s bulkiness may hinder reactions requiring planar transition states (e.g., S<sub>N</sub>2) .
-
Solubility : Limited solubility in polar solvents necessitates phase-transfer catalysts .
[Sources: 1, 3, 5, 7, 8, 10, 11, 12]
Scientific Research Applications
Organic Synthesis
DEOM is extensively used as a building block in organic synthesis, particularly in the preparation of various derivatives and complex molecules. Its utility arises from its ability to undergo reactions such as:
- Alkylation: DEOM can serve as a precursor for the synthesis of higher aliphatic compounds through alkylation reactions.
- Condensation Reactions: It participates in condensation reactions to form larger molecular frameworks, which are essential in the synthesis of pharmaceuticals.
Case Study: Alkylation Reactions
In a study published in the Journal of Organic Chemistry, researchers utilized DEOM for the synthesis of alkylated malonates, demonstrating its efficacy in generating diverse alkyl chains suitable for further functionalization .
Pharmaceutical Applications
This compound has shown promise in pharmaceutical applications, particularly as an intermediate in the synthesis of bioactive compounds. Its derivatives have been investigated for their potential therapeutic effects.
- Anticancer Agents: Some studies have focused on DEOM derivatives that exhibit anticancer properties. For instance, modifications to the malonate structure have led to compounds that inhibit tumor growth in vitro.
Case Study: Anticancer Activity
A research paper published in Medicinal Chemistry highlighted the synthesis of this compound derivatives that demonstrated significant cytotoxicity against various cancer cell lines, indicating potential for development into therapeutic agents .
Material Science
In material science, DEOM is utilized as a plasticizer and additive in polymer formulations. Its incorporation enhances the flexibility and durability of polymers.
- Polymer Blends: DEOM has been used to modify polyvinyl chloride (PVC) and other polymers, improving their mechanical properties without compromising thermal stability.
Data Table: Properties of DEOM-modified Polymers
Property | PVC (Control) | PVC + DEOM |
---|---|---|
Flexibility | Low | High |
Thermal Stability | Moderate | High |
Tensile Strength | Moderate | High |
Environmental Applications
Recent studies have explored the potential use of DEOM in environmental applications, particularly in biodegradable materials. Research indicates that incorporating DEOM into biodegradable plastics can enhance their performance while maintaining eco-friendliness.
Case Study: Biodegradable Plastics
A study conducted by environmental scientists demonstrated that blending DEOM with polylactic acid (PLA) resulted in improved degradation rates under composting conditions, making it a viable candidate for sustainable packaging solutions .
Mechanism of Action
The mechanism of action of diethyl octylmalonate involves its reactivity as a malonate ester. The compound can undergo nucleophilic substitution reactions, where the methylene group between the ester groups acts as a nucleophile.
Comparison with Similar Compounds
Comparison with Similar Compounds
The following table and analysis compare diethyl octylmalonate with structurally related malonate esters, focusing on molecular properties, applications, and key differences.
Table 1: Comparative Analysis of Diethyl Malonate Esters
Key Comparisons
Structural and Physical Properties Octyl vs. Shorter Chains: this compound’s octyl chain increases molecular weight (244.33 g/mol) and hydrophobicity compared to diethyl malonate (160.17 g/mol). This enhances its compatibility with non-polar matrices, making it suitable for plasticizers or lubricants . Benzyl vs. Alkyl Groups: Diethyl benzylmalonate’s aromatic benzyl group (250.29 g/mol) introduces rigidity and π-π interactions, favoring crystallinity in pharmaceuticals. In contrast, the flexible octyl chain in this compound reduces melting points and improves solubility in oils .
Reactivity and Applications
- Diallyl Substitution : Diethyl diallylmalonate’s dual allyl groups (240.29 g/mol) enable radical polymerization or thiol-ene reactions, critical in creating crosslinked polymers. The octyl variant lacks this reactivity but offers better thermal stability .
- Oxo Functionality : Diethyl 2-oxomalonate (174.15 g/mol) undergoes decarboxylation upon heating, releasing CO₂. This reactivity is absent in this compound, which is more stable due to its alkyl substituent .
Safety and Handling
- Diethyl malonate derivatives generally require standard laboratory precautions (e.g., ventilation, gloves). For example, diethyl malonate’s safety data sheet (CAS 105-53-3) highlights flammability and eye irritation risks . Octylmalonate’s longer alkyl chain may reduce volatility but could pose bioaccumulation concerns .
Research Findings and Trends
- Synthetic Utility: Diethyl malonate esters are pivotal in multicomponent reactions. For instance, diethyl ethoxymethylenemalonate (CAS 87-13-8) is a key reagent in synthesizing heterocycles like quinolones . Octylmalonate’s applications remain underexplored but could mirror uses in specialty polymers or agrochemicals.
- Thermodynamic Data: Excess molar enthalpies of diethyl malonate with solvents (e.g., 1-butanol, heptane) have been measured, aiding in industrial process optimization . Similar studies for octylmalonate would clarify its mixing behaviors.
Biological Activity
Diethyl octylmalonate (DEOM) is a chemical compound that has garnered attention for its potential biological activities. This article explores its biological properties, mechanisms of action, and relevant case studies, providing a comprehensive overview of the current understanding of DEOM's effects on biological systems.
This compound is classified as a malonate ester, with the molecular formula and a molar mass of 284.39 g/mol. Its structure consists of two ethyl groups and an octyl chain attached to a malonate backbone, which contributes to its lipophilicity and potential interactions with biological membranes.
Research has indicated that DEOM may exert its biological effects through several mechanisms:
- Inhibition of Mitochondrial Function : DEOM has been shown to inhibit mitochondrial succinate transport, leading to the accumulation of succinate within cells. This disruption can affect cellular respiration and energy production, which may have implications in metabolic diseases and cancer treatment .
- Antioxidant Activity : Some studies suggest that DEOM may possess antioxidant properties, potentially mitigating oxidative stress in various cellular contexts. The specific pathways through which DEOM acts as an antioxidant are still under investigation .
- Modulation of Immune Responses : DEOM has been implicated in modulating immune responses, particularly in the context of inflammation. Its effects on metabolic pathways in immune cells may influence their polarization and function during inflammatory responses .
Biological Activity
The biological activity of this compound can be summarized as follows:
Case Studies
-
Mitochondrial Dysfunction in Disease Models :
A study demonstrated that DEOM's inhibition of mitochondrial succinate transport led to increased levels of reactive oxygen species (ROS) in macrophages infected with Mycobacterium tuberculosis. This suggests that DEOM could play a role in exacerbating oxidative stress during infections, highlighting its dual role as both a potential therapeutic agent and a compound that may contribute to pathology under certain conditions . -
Effects on Immune Cell Metabolism :
Research involving human mesenchymal stem cells (hMSCs) indicated that treatment with DEOM altered their metabolic profile, enhancing indoleamine 2,3-dioxygenase (IDO) production under hypoxic conditions. This finding points to the compound's potential in modulating immune responses through metabolic reprogramming, which could have therapeutic implications in immunotherapy . -
Antioxidant Studies :
In vitro studies have suggested that DEOM may exhibit antioxidant properties by scavenging free radicals and reducing lipid peroxidation. These effects could be beneficial in protecting against oxidative damage in various cellular models .
Q & A
Basic Research Questions
Q. What synthetic strategies are effective for preparing diethyl octylmalonate, and how can reaction conditions be optimized?
this compound can be synthesized via alkylation of diethyl malonate enolates. The reaction typically involves deprotonating diethyl malonate with a strong base (e.g., NaOEt or LDA) to form the enolate, followed by alkylation with an octyl halide (e.g., 1-bromooctane). Key optimization parameters include:
- Base selection : Stronger bases (e.g., LDA) enhance enolate formation but may reduce selectivity due to side reactions .
- Solvent polarity : Polar aprotic solvents (e.g., DMF) improve electrophile-enolate interactions, while ethereal solvents (e.g., THF) favor kinetic control .
- Temperature : Lower temperatures (−78°C to 0°C) minimize decarboxylation side reactions . Post-reaction purification via fractional distillation or column chromatography is recommended to isolate the product.
Q. How can the structure of this compound be confirmed using spectroscopic methods?
- NMR :
- ¹H NMR : Look for ethyl ester protons (δ 1.2–1.4 ppm, triplet; δ 4.1–4.3 ppm, quartet) and octyl chain protons (δ 0.8–1.6 ppm, multiplet). The central malonate CH₂ group appears as a singlet (δ 3.3–3.5 ppm) .
- ¹³C NMR : Confirm ester carbonyls (δ 165–170 ppm) and the malonate carbon (δ 45–50 ppm) .
Advanced Research Questions
Q. How does the octyl substituent influence the reactivity and selectivity of this compound in enolate alkylation?
The octyl group introduces steric hindrance, which:
- Reduces electrophilic attack rates : Bulky substituents slow alkylation kinetics, favoring thermodynamic control over products .
- Impacts regioselectivity : In unsymmetrical systems, steric effects may direct alkylation to less hindered positions. Computational modeling (e.g., DFT) can predict regiochemical outcomes .
- Modifies solubility : The hydrophobic octyl chain enhances solubility in nonpolar solvents, complicating aqueous workup .
Q. What analytical challenges arise when quantifying this compound in complex reaction mixtures, and how can they be addressed?
- Co-elution in chromatography : Use orthogonal techniques (e.g., HPLC with UV/Vis and MS detection) to resolve overlapping peaks .
- Matrix interference : Employ internal standards (e.g., deuterated analogs) for accurate quantification in GC-MS or LC-MS .
- Degradation products : Monitor for decarboxylation byproducts (e.g., diethyl succinate derivatives) via tandem MS .
Q. What mechanistic insights explain the stability of this compound under acidic vs. basic conditions?
- Acidic conditions : Protonation of the ester carbonyls increases electrophilicity, accelerating hydrolysis to malonic acid and octanol. The reaction follows a nucleophilic acyl substitution mechanism .
- Basic conditions : Saponification of esters occurs via hydroxide attack, forming carboxylates. The octyl group’s hydrophobicity slows aqueous-phase hydrolysis compared to shorter-chain analogs . Kinetic studies (e.g., Arrhenius plots) can quantify degradation rates .
Q. Methodological Considerations
Q. How can computational chemistry tools aid in predicting the physicochemical properties of this compound?
- Molecular dynamics (MD) simulations : Predict solubility parameters and partition coefficients (logP) .
- Density Functional Theory (DFT) : Calculate bond dissociation energies (BDEs) to assess thermal stability and reactive sites .
- QSAR models : Relate structural descriptors (e.g., molar volume, polar surface area) to experimental data (e.g., boiling point, viscosity) .
Q. What strategies mitigate side reactions during this compound’s use in multi-step syntheses?
- Protecting groups : Temporarily block reactive malonate hydrogens with silyl groups (e.g., TMSCl) to prevent undesired alkylation .
- Low-temperature conditions : Maintain reactions below −20°C to suppress decarboxylation .
- Catalytic additives : Use phase-transfer catalysts (e.g., tetrabutylammonium bromide) to enhance interfacial reactivity in biphasic systems .
Properties
IUPAC Name |
diethyl 2-octylpropanedioate | |
---|---|---|
Source | PubChem | |
URL | https://pubchem.ncbi.nlm.nih.gov | |
Description | Data deposited in or computed by PubChem | |
InChI |
InChI=1S/C15H28O4/c1-4-7-8-9-10-11-12-13(14(16)18-5-2)15(17)19-6-3/h13H,4-12H2,1-3H3 | |
Source | PubChem | |
URL | https://pubchem.ncbi.nlm.nih.gov | |
Description | Data deposited in or computed by PubChem | |
InChI Key |
YZYBVYGISZANLI-UHFFFAOYSA-N | |
Source | PubChem | |
URL | https://pubchem.ncbi.nlm.nih.gov | |
Description | Data deposited in or computed by PubChem | |
Canonical SMILES |
CCCCCCCCC(C(=O)OCC)C(=O)OCC | |
Source | PubChem | |
URL | https://pubchem.ncbi.nlm.nih.gov | |
Description | Data deposited in or computed by PubChem | |
Molecular Formula |
C15H28O4 | |
Source | PubChem | |
URL | https://pubchem.ncbi.nlm.nih.gov | |
Description | Data deposited in or computed by PubChem | |
DSSTOX Substance ID |
DTXSID50291209 | |
Record name | Diethyl octylmalonate | |
Source | EPA DSSTox | |
URL | https://comptox.epa.gov/dashboard/DTXSID50291209 | |
Description | DSSTox provides a high quality public chemistry resource for supporting improved predictive toxicology. | |
Molecular Weight |
272.38 g/mol | |
Source | PubChem | |
URL | https://pubchem.ncbi.nlm.nih.gov | |
Description | Data deposited in or computed by PubChem | |
CAS No. |
1472-85-1 | |
Record name | Diethyl octylmalonate | |
Source | DTP/NCI | |
URL | https://dtp.cancer.gov/dtpstandard/servlet/dwindex?searchtype=NSC&outputformat=html&searchlist=74080 | |
Description | The NCI Development Therapeutics Program (DTP) provides services and resources to the academic and private-sector research communities worldwide to facilitate the discovery and development of new cancer therapeutic agents. | |
Explanation | Unless otherwise indicated, all text within NCI products is free of copyright and may be reused without our permission. Credit the National Cancer Institute as the source. | |
Record name | Diethyl octylmalonate | |
Source | EPA DSSTox | |
URL | https://comptox.epa.gov/dashboard/DTXSID50291209 | |
Description | DSSTox provides a high quality public chemistry resource for supporting improved predictive toxicology. | |
Synthesis routes and methods I
Procedure details
Synthesis routes and methods II
Procedure details
Synthesis routes and methods III
Procedure details
Disclaimer and Information on In-Vitro Research Products
Please be aware that all articles and product information presented on BenchChem are intended solely for informational purposes. The products available for purchase on BenchChem are specifically designed for in-vitro studies, which are conducted outside of living organisms. In-vitro studies, derived from the Latin term "in glass," involve experiments performed in controlled laboratory settings using cells or tissues. It is important to note that these products are not categorized as medicines or drugs, and they have not received approval from the FDA for the prevention, treatment, or cure of any medical condition, ailment, or disease. We must emphasize that any form of bodily introduction of these products into humans or animals is strictly prohibited by law. It is essential to adhere to these guidelines to ensure compliance with legal and ethical standards in research and experimentation.