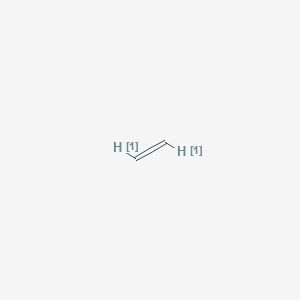
(E)-1,2-Dideuteroethylene
Overview
Description
Synthesis Analysis
The synthesis of (E)-1,2-Dideuteroethylene involves reducing dideuteroacetylene with chromous chloride solution and copper-activated zinc dust for the trans and cis isomers, respectively. This process yields polydideuteroethylenes with stereoregulated structures, demonstrating significant advancements in polymer chemistry through the utilization of Ziegler catalysts for polymerizing these isomers (Ikeda, Yamamoto, & Tanaka, 1963).
Molecular Structure Analysis
The molecular geometry and vapor pressure of isotopic molecules, including (E)-1,2-Dideuteroethylene, have been investigated to understand their structural differences. It has been found that differences in molecular geometry correlate with variations in vapor pressure, providing insights into hindered rotation in the liquid phase of these molecules (Bigeleisen, Ribnikar, & Hook, 1963).
Chemical Reactions and Properties
Research on the Cd(3P1)-photosensitized isomerization of dideuteroethylene over temperatures ranging from 275–350 °C reveals the formation of cis-1,2- and asym-1,1-dideuteroethylenes as primary products. This study elucidates the mechanistic pathways of isomerization, highlighting the minor products ethane, acetylene, and n-butane (Tsunashima, Hirokami, & Sato, 1968).
Physical Properties Analysis
The spin-lattice relaxation times of trans-1,2-dichloroethylene indicate a liquid-liquid phase transition at -16°C, segregating its liquid state into two distinct phases, L1 and L2. This discovery of a mesomorphic state in trans-1,2-dichloroethylene, akin to a nematic state, sheds light on the unique behavior of molecular motions in different liquid states (Kawanishi, Sasuga, & Takehisa, 1982).
Chemical Properties Analysis
The mass spectra of deuteroethylenes have provided valuable insights into the molecular structure and reactivity of these compounds, elucidating the isotopic effects on their chemical behavior. This research has been crucial for understanding the fundamental properties and reactions of dideuteroethylene and its derivatives (Dibeler, Mohler, & Hemptinne, 1954).
Mechanism of Action
- Unfortunately, specific primary targets for 1,2-diprotioethene are not well-documented in the available literature. However, it belongs to the class of organic compounds known as dialkyl ethers . These compounds contain the dialkyl ether functional group (ROR’), where R and R’ are alkyl groups.
Target of Action
properties
IUPAC Name |
1,2-diprotioethene | |
---|---|---|
Source | PubChem | |
URL | https://pubchem.ncbi.nlm.nih.gov | |
Description | Data deposited in or computed by PubChem | |
InChI |
InChI=1S/C2H4/c1-2/h1-2H2/i1H,2H | |
Source | PubChem | |
URL | https://pubchem.ncbi.nlm.nih.gov | |
Description | Data deposited in or computed by PubChem | |
InChI Key |
VGGSQFUCUMXWEO-JCIKQZAVSA-N | |
Source | PubChem | |
URL | https://pubchem.ncbi.nlm.nih.gov | |
Description | Data deposited in or computed by PubChem | |
Canonical SMILES |
C=C | |
Source | PubChem | |
URL | https://pubchem.ncbi.nlm.nih.gov | |
Description | Data deposited in or computed by PubChem | |
Isomeric SMILES |
[1H]C=C[1H] | |
Source | PubChem | |
URL | https://pubchem.ncbi.nlm.nih.gov | |
Description | Data deposited in or computed by PubChem | |
Molecular Formula |
C2H4 | |
Source | PubChem | |
URL | https://pubchem.ncbi.nlm.nih.gov | |
Description | Data deposited in or computed by PubChem | |
Molecular Weight |
28.05 g/mol | |
Source | PubChem | |
URL | https://pubchem.ncbi.nlm.nih.gov | |
Description | Data deposited in or computed by PubChem | |
Product Name |
(E)-1,2-Dideuteroethylene | |
CAS RN |
1517-53-9 | |
Record name | (E)-1,2-Dideuteroethylene | |
Source | ChemIDplus | |
URL | https://pubchem.ncbi.nlm.nih.gov/substance/?source=chemidplus&sourceid=0001517539 | |
Description | ChemIDplus is a free, web search system that provides access to the structure and nomenclature authority files used for the identification of chemical substances cited in National Library of Medicine (NLM) databases, including the TOXNET system. | |
Synthesis routes and methods I
Procedure details
Synthesis routes and methods II
Procedure details
Synthesis routes and methods III
Procedure details
Synthesis routes and methods IV
Procedure details
Disclaimer and Information on In-Vitro Research Products
Please be aware that all articles and product information presented on BenchChem are intended solely for informational purposes. The products available for purchase on BenchChem are specifically designed for in-vitro studies, which are conducted outside of living organisms. In-vitro studies, derived from the Latin term "in glass," involve experiments performed in controlled laboratory settings using cells or tissues. It is important to note that these products are not categorized as medicines or drugs, and they have not received approval from the FDA for the prevention, treatment, or cure of any medical condition, ailment, or disease. We must emphasize that any form of bodily introduction of these products into humans or animals is strictly prohibited by law. It is essential to adhere to these guidelines to ensure compliance with legal and ethical standards in research and experimentation.