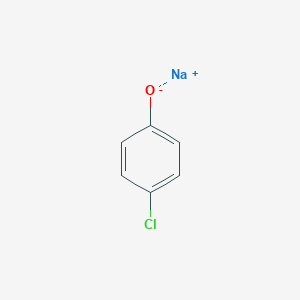
Sodium 4-chlorophenolate
Overview
Description
Sodium 4-chlorophenolate is a chemical compound with the molecular formula C₆H₄ClNaO. It is the sodium salt of 4-chlorophenol and is commonly used in various industrial and pharmaceutical applications. This compound is known for its antibacterial and antifungal properties, making it useful in a range of applications from disinfectants to preservatives .
Preparation Methods
Synthetic Routes and Reaction Conditions: Sodium 4-chlorophenolate is typically synthesized by the reaction of 4-chlorophenol with sodium hydroxide. The reaction can be represented as follows: [ \text{C}_6\text{H}_4\text{ClOH} + \text{NaOH} \rightarrow \text{C}_6\text{H}_4\text{ClONa} + \text{H}_2\text{O} ] In this reaction, 4-chlorophenol is dissolved in an alkaline solution, and sodium hydroxide is added to form this compound .
Industrial Production Methods: In industrial settings, the production of this compound involves the use of large-scale reactors where 4-chlorophenol and sodium hydroxide are mixed under controlled conditions. The reaction is typically carried out at room temperature, and the product is purified through crystallization or other separation techniques .
Types of Reactions:
Oxidation: this compound can undergo oxidation reactions to form various oxidation products.
Reduction: It can be reduced under specific conditions to yield different reduced forms.
Substitution: This compound is known to undergo nucleophilic substitution reactions, where the chlorine atom can be replaced by other nucleophiles.
Common Reagents and Conditions:
Oxidation: Common oxidizing agents include potassium permanganate and hydrogen peroxide.
Reduction: Reducing agents such as sodium borohydride can be used.
Substitution: Nucleophiles like hydroxide ions or amines are commonly used in substitution reactions.
Major Products Formed:
Oxidation: Various chlorinated phenolic compounds.
Reduction: Reduced phenolic derivatives.
Substitution: Substituted phenolic compounds with different functional groups.
Scientific Research Applications
Sodium 4-chlorophenolate has a wide range of applications in scientific research:
Chemistry: It is used as a reagent in organic synthesis, particularly in the preparation of other chlorinated phenolic compounds.
Biology: It is employed in studies involving bacterial and fungal inhibition due to its antimicrobial properties.
Medicine: Historically, it has been used as a topical antiseptic in ointments and as a local antibacterial agent in root canal therapy.
Industry: It is used as a fungicide, preservative, and antibacterial agent in the pharmaceutical and pesticide industries .
Mechanism of Action
The mechanism of action of sodium 4-chlorophenolate involves its interaction with microbial cell membranes. It disrupts the cell membrane integrity, leading to leakage of cellular contents and ultimately cell death. This compound targets various molecular pathways involved in cell membrane synthesis and maintenance, making it effective against a broad spectrum of bacteria and fungi .
Comparison with Similar Compounds
- Sodium 2-chlorophenolate
- Sodium 3-chlorophenolate
- Sodium 4-bromophenolate
Comparison: Sodium 4-chlorophenolate is unique due to its specific chlorine substitution at the para position, which imparts distinct chemical and biological properties. Compared to sodium 2-chlorophenolate and sodium 3-chlorophenolate, the para-substitution in this compound results in different reactivity and antimicrobial efficacy. Sodium 4-bromophenolate, on the other hand, has a bromine atom instead of chlorine, which can lead to variations in its chemical behavior and applications .
Properties
CAS No. |
1193-00-6 |
---|---|
Molecular Formula |
C6H5ClNaO |
Molecular Weight |
151.54 g/mol |
IUPAC Name |
sodium;4-chlorophenolate |
InChI |
InChI=1S/C6H5ClO.Na/c7-5-1-3-6(8)4-2-5;/h1-4,8H; |
InChI Key |
MXJBIALFHGDUPE-UHFFFAOYSA-N |
SMILES |
C1=CC(=CC=C1[O-])Cl.[Na+] |
Isomeric SMILES |
C1=CC(=CC=C1[O-])Cl.[Na+] |
Canonical SMILES |
C1=CC(=CC=C1O)Cl.[Na] |
1193-00-6 | |
Related CAS |
106-48-9 (Parent) |
Origin of Product |
United States |
Synthesis routes and methods
Procedure details
Retrosynthesis Analysis
AI-Powered Synthesis Planning: Our tool employs the Template_relevance Pistachio, Template_relevance Bkms_metabolic, Template_relevance Pistachio_ringbreaker, Template_relevance Reaxys, Template_relevance Reaxys_biocatalysis model, leveraging a vast database of chemical reactions to predict feasible synthetic routes.
One-Step Synthesis Focus: Specifically designed for one-step synthesis, it provides concise and direct routes for your target compounds, streamlining the synthesis process.
Accurate Predictions: Utilizing the extensive PISTACHIO, BKMS_METABOLIC, PISTACHIO_RINGBREAKER, REAXYS, REAXYS_BIOCATALYSIS database, our tool offers high-accuracy predictions, reflecting the latest in chemical research and data.
Strategy Settings
Precursor scoring | Relevance Heuristic |
---|---|
Min. plausibility | 0.01 |
Model | Template_relevance |
Template Set | Pistachio/Bkms_metabolic/Pistachio_ringbreaker/Reaxys/Reaxys_biocatalysis |
Top-N result to add to graph | 6 |
Feasible Synthetic Routes
Disclaimer and Information on In-Vitro Research Products
Please be aware that all articles and product information presented on BenchChem are intended solely for informational purposes. The products available for purchase on BenchChem are specifically designed for in-vitro studies, which are conducted outside of living organisms. In-vitro studies, derived from the Latin term "in glass," involve experiments performed in controlled laboratory settings using cells or tissues. It is important to note that these products are not categorized as medicines or drugs, and they have not received approval from the FDA for the prevention, treatment, or cure of any medical condition, ailment, or disease. We must emphasize that any form of bodily introduction of these products into humans or animals is strictly prohibited by law. It is essential to adhere to these guidelines to ensure compliance with legal and ethical standards in research and experimentation.