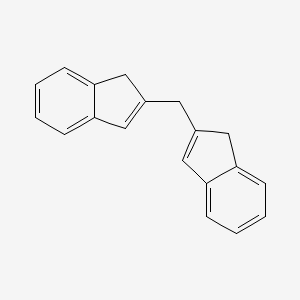
Bis(2-indenyl)methane
Overview
Description
Bis(2-indenyl)methane is an organic compound that features two indenyl groups connected by a methylene bridge Indenyl groups are derived from indene, a bicyclic hydrocarbon consisting of a benzene ring fused to a cyclopentadiene ring
Preparation Methods
Synthetic Routes and Reaction Conditions: The synthesis of bis(2-indenyl)methane typically involves the reaction of indene with formaldehyde in the presence of an acid catalyst. The reaction proceeds via electrophilic aromatic substitution, where the formaldehyde acts as the electrophile, and the indene acts as the nucleophile. The general reaction conditions include:
Catalyst: Acidic catalysts such as sulfuric acid or hydrochloric acid.
Temperature: Moderate temperatures, typically around 50-70°C.
Solvent: Non-polar solvents like toluene or dichloromethane.
Industrial Production Methods: While specific industrial production methods for this compound are not well-documented, the general approach would involve scaling up the laboratory synthesis. This would include optimizing reaction conditions for higher yields and purity, using continuous flow reactors, and employing more efficient separation and purification techniques.
Types of Reactions:
Oxidation: this compound can undergo oxidation reactions to form various oxygenated derivatives. Common oxidizing agents include potassium permanganate and chromium trioxide.
Reduction: Reduction reactions can convert this compound to its corresponding dihydro derivatives. Typical reducing agents include lithium aluminum hydride and sodium borohydride.
Substitution: Electrophilic substitution reactions can introduce various functional groups into the indenyl rings. Common reagents include halogens (chlorine, bromine) and nitrating agents.
Common Reagents and Conditions:
Oxidation: Potassium permanganate in an aqueous medium at room temperature.
Reduction: Lithium aluminum hydride in anhydrous ether at low temperatures.
Substitution: Halogenation using bromine in carbon tetrachloride at room temperature.
Major Products:
Oxidation: Indenone derivatives.
Reduction: Dihydroindenyl derivatives.
Substitution: Halogenated or nitrated this compound.
Scientific Research Applications
Bis(2-indenyl)methane has several applications in scientific research:
Chemistry: Used as a ligand in organometallic chemistry for the synthesis of metal complexes.
Materials Science:
Biology and Medicine: Investigated for its potential biological activity, including anti-cancer and anti-inflammatory properties.
Industry: Used in the synthesis of polymers and as a precursor for various chemical compounds.
Mechanism of Action
The mechanism by which bis(2-indenyl)methane exerts its effects depends on its specific application. In organometallic chemistry, it acts as a ligand, coordinating to metal centers and influencing their reactivity and stability. In biological systems, its mechanism of action may involve interactions with cellular proteins and enzymes, leading to modulation of signaling pathways and gene expression.
Comparison with Similar Compounds
Bis(indolyl)methane: Similar structure but with indole groups instead of indenyl groups.
Bis(pyrazolyl)methane: Contains pyrazole rings instead of indenyl rings.
Bis(phenyl)methane: Features phenyl groups instead of indenyl groups.
Uniqueness: Bis(2-indenyl)methane is unique due to the presence of indenyl groups, which confer distinct electronic and steric properties compared to other bis(aryl)methanes. This uniqueness makes it valuable in specific applications, particularly in the field of organometallic chemistry and materials science.
Properties
IUPAC Name |
2-(1H-inden-2-ylmethyl)-1H-indene | |
---|---|---|
Details | Computed by Lexichem TK 2.7.0 (PubChem release 2021.05.07) | |
Source | PubChem | |
URL | https://pubchem.ncbi.nlm.nih.gov | |
Description | Data deposited in or computed by PubChem | |
InChI |
InChI=1S/C19H16/c1-2-6-17-11-14(10-16(17)5-1)9-15-12-18-7-3-4-8-19(18)13-15/h1-8,10,12H,9,11,13H2 | |
Details | Computed by InChI 1.0.6 (PubChem release 2021.05.07) | |
Source | PubChem | |
URL | https://pubchem.ncbi.nlm.nih.gov | |
Description | Data deposited in or computed by PubChem | |
InChI Key |
VBDPXNNRCIXHFT-UHFFFAOYSA-N | |
Details | Computed by InChI 1.0.6 (PubChem release 2021.05.07) | |
Source | PubChem | |
URL | https://pubchem.ncbi.nlm.nih.gov | |
Description | Data deposited in or computed by PubChem | |
Canonical SMILES |
C1C2=CC=CC=C2C=C1CC3=CC4=CC=CC=C4C3 | |
Details | Computed by OEChem 2.3.0 (PubChem release 2021.05.07) | |
Source | PubChem | |
URL | https://pubchem.ncbi.nlm.nih.gov | |
Description | Data deposited in or computed by PubChem | |
Molecular Formula |
C19H16 | |
Details | Computed by PubChem 2.1 (PubChem release 2021.05.07) | |
Source | PubChem | |
URL | https://pubchem.ncbi.nlm.nih.gov | |
Description | Data deposited in or computed by PubChem | |
Molecular Weight |
244.3 g/mol | |
Details | Computed by PubChem 2.1 (PubChem release 2021.05.07) | |
Source | PubChem | |
URL | https://pubchem.ncbi.nlm.nih.gov | |
Description | Data deposited in or computed by PubChem | |
Synthesis routes and methods I
Procedure details
Synthesis routes and methods II
Procedure details
Retrosynthesis Analysis
AI-Powered Synthesis Planning: Our tool employs the Template_relevance Pistachio, Template_relevance Bkms_metabolic, Template_relevance Pistachio_ringbreaker, Template_relevance Reaxys, Template_relevance Reaxys_biocatalysis model, leveraging a vast database of chemical reactions to predict feasible synthetic routes.
One-Step Synthesis Focus: Specifically designed for one-step synthesis, it provides concise and direct routes for your target compounds, streamlining the synthesis process.
Accurate Predictions: Utilizing the extensive PISTACHIO, BKMS_METABOLIC, PISTACHIO_RINGBREAKER, REAXYS, REAXYS_BIOCATALYSIS database, our tool offers high-accuracy predictions, reflecting the latest in chemical research and data.
Strategy Settings
Precursor scoring | Relevance Heuristic |
---|---|
Min. plausibility | 0.01 |
Model | Template_relevance |
Template Set | Pistachio/Bkms_metabolic/Pistachio_ringbreaker/Reaxys/Reaxys_biocatalysis |
Top-N result to add to graph | 6 |
Feasible Synthetic Routes
Disclaimer and Information on In-Vitro Research Products
Please be aware that all articles and product information presented on BenchChem are intended solely for informational purposes. The products available for purchase on BenchChem are specifically designed for in-vitro studies, which are conducted outside of living organisms. In-vitro studies, derived from the Latin term "in glass," involve experiments performed in controlled laboratory settings using cells or tissues. It is important to note that these products are not categorized as medicines or drugs, and they have not received approval from the FDA for the prevention, treatment, or cure of any medical condition, ailment, or disease. We must emphasize that any form of bodily introduction of these products into humans or animals is strictly prohibited by law. It is essential to adhere to these guidelines to ensure compliance with legal and ethical standards in research and experimentation.