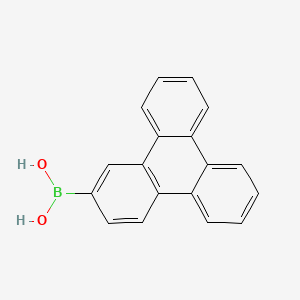
Triphenylen-2-ylboronic acid
Overview
Description
Triphenylen-2-ylboronic acid, also known as boronic acid, 2-triphenylenyl-, is an organic compound with the chemical formula C18H13BO2. It is a derivative of benzimidazole ligands and is characterized by its white or light yellow crystalline form. This compound is notable for its relatively high melting and boiling points and its good solubility in organic solvents .
Mechanism of Action
Target of Action
Triphenylen-2-ylboronic acid is a chemical compound with the molecular formula C18H13BO2 The primary targets of this compound are not explicitly mentioned in the available literature
Biochemical Pathways
It has been used in the development of organic polymers with ultralong room-temperature phosphorescence (rtp) lifetimes . This suggests that it may play a role in energy transfer processes within these materials.
Pharmacokinetics
Its boiling point is 565.5±33.0 °C at 760 mmHg , and its density is 1.3±0.1 g/cm3 . These properties could influence its bioavailability and pharmacokinetics.
Result of Action
Its use in the development of organic polymers with ultralong rtp lifetimes suggests that it may influence the energy transfer processes within these materials .
Action Environment
The action of this compound can be influenced by various environmental factors. For instance, its stability and efficacy could be affected by temperature, as it should be stored under inert gas (nitrogen or Argon) at 2-8°C
Biochemical Analysis
Biochemical Properties
Triphenylen-2-ylboronic acid plays a crucial role in biochemical reactions, particularly as a ligand in metal-catalyzed processes. It interacts with various enzymes and proteins, facilitating important catalytic reactions. For instance, it is often used in Suzuki-Miyaura cross-coupling reactions, where it interacts with palladium catalysts to form carbon-carbon bonds . The nature of these interactions involves the coordination of the boronic acid group with the metal center, enhancing the reactivity and selectivity of the catalytic process.
Cellular Effects
This compound influences various cellular processes, including cell signaling pathways, gene expression, and cellular metabolism. It has been observed to affect the activity of certain kinases and phosphatases, thereby modulating signal transduction pathways . Additionally, it can influence gene expression by interacting with transcription factors and other regulatory proteins, leading to changes in cellular metabolism and function.
Molecular Mechanism
At the molecular level, this compound exerts its effects through binding interactions with biomolecules. It can act as an enzyme inhibitor or activator, depending on the specific biochemical context. For example, it has been shown to inhibit certain proteases by forming a stable complex with the enzyme’s active site . This inhibition can lead to changes in gene expression and cellular responses, highlighting the compound’s potential as a therapeutic agent.
Temporal Effects in Laboratory Settings
In laboratory settings, the effects of this compound can change over time due to its stability and degradation properties. The compound is relatively stable under inert gas conditions (such as nitrogen or argon) at temperatures between 2-8°C . Prolonged exposure to air and moisture can lead to degradation, affecting its efficacy in biochemical assays. Long-term studies have shown that this compound can have sustained effects on cellular function, particularly in in vitro settings .
Dosage Effects in Animal Models
The effects of this compound vary with different dosages in animal models. At low doses, the compound has been observed to enhance certain metabolic pathways without causing significant toxicity . At higher doses, it can exhibit toxic effects, including oxidative stress and cellular damage. These threshold effects highlight the importance of careful dosage optimization in preclinical studies.
Metabolic Pathways
This compound is involved in several metabolic pathways, interacting with enzymes and cofactors to modulate metabolic flux. It has been shown to influence the activity of enzymes involved in the synthesis of aromatic amino acids and other secondary metabolites . These interactions can lead to changes in metabolite levels and overall metabolic homeostasis.
Transport and Distribution
Within cells and tissues, this compound is transported and distributed through interactions with specific transporters and binding proteins. These interactions facilitate its localization to specific cellular compartments, where it can exert its biochemical effects . The compound’s distribution is influenced by its physicochemical properties, including its solubility and affinity for cellular membranes.
Subcellular Localization
This compound exhibits specific subcellular localization patterns, which can affect its activity and function. It is often directed to particular compartments or organelles through targeting signals and post-translational modifications . For example, it may localize to the nucleus or mitochondria, where it can interact with key regulatory proteins and enzymes to modulate cellular processes.
Preparation Methods
The preparation of Triphenylen-2-ylboronic acid is typically achieved through metal-catalyzed coupling reactions. One common method involves the base-catalyzed reaction of a borate ester with an aromatic aldehyde. The reaction conditions often require precise control to ensure high purity and yield . For example, a specific synthetic route involves the use of n-butyllithium in tetrahydrofuran and hexane at low temperatures, followed by the addition of trimethoxyborane and subsequent warming to room temperature .
Chemical Reactions Analysis
Triphenylen-2-ylboronic acid undergoes various types of chemical reactions, including:
Oxidation: It can be oxidized under specific conditions to form corresponding boronic acids.
Reduction: It can be reduced to form boronic esters.
Common reagents used in these reactions include palladium catalysts, bases like potassium phosphate, and solvents such as toluene and water. The major products formed from these reactions are often intermediates for further organic synthesis .
Scientific Research Applications
Triphenylen-2-ylboronic acid has a wide range of applications in scientific research:
Chemistry: It is used as a ligand in metal-catalyzed reactions, playing a crucial role in asymmetric synthesis and cross-coupling reactions.
Biology: It is utilized in the synthesis of fluorescent dyes and photosensitive materials, which are important in biological imaging and diagnostics.
Medicine: It serves as an intermediate in the synthesis of pharmaceutical compounds.
Industry: It is used in the production of fluorine intermediates and other specialty chemicals
Comparison with Similar Compounds
Triphenylen-2-ylboronic acid can be compared with other boronic acids such as phenylboronic acid and naphthylboronic acid. While all these compounds serve as ligands in metal-catalyzed reactions, this compound is unique due to its specific structure, which provides distinct electronic properties and reactivity. Similar compounds include:
- Phenylboronic acid
- Naphthylboronic acid
- Benzothiophen-2-ylboronic acid .
These compounds share similar applications but differ in their structural and electronic characteristics, which influence their reactivity and suitability for specific reactions.
Biological Activity
Triphenylen-2-ylboronic acid is a boronic acid derivative that has garnered interest in various fields, particularly in biological and materials science. This compound exhibits unique properties that make it suitable for applications in organic synthesis, biological imaging, and the development of advanced materials. This article provides a comprehensive overview of the biological activity of this compound, including its mechanisms of action, biochemical pathways, and potential applications.
Molecular Formula: C₁₈H₁₃BO₂
Boiling Point: 565.5 ± 33.0 °C at 760 mmHg
Density: 1.3 ± 0.1 g/cm³
This compound is characterized by its ability to form reversible covalent bonds with various functional groups, such as hydroxyl (-OH) and amine (-NH₂) groups. This property is particularly valuable in Suzuki-Miyaura coupling reactions, which are fundamental in organic synthesis for forming carbon-carbon bonds .
Target of Action
The compound acts primarily through its interactions with enzymes and proteins within biological systems. It can function as an enzyme inhibitor or activator depending on the specific biochemical context. For instance, it has been shown to inhibit certain proteases by forming stable complexes with their active sites.
Biochemical Pathways
This compound plays a crucial role in several biochemical pathways:
- Cell Signaling: It influences cell signaling pathways by modulating the activity of kinases and phosphatases, thereby affecting gene expression and cellular metabolism.
- Energy Transfer Processes: The compound has been utilized in developing organic polymers with ultralong room-temperature phosphorescence (RTP), indicating its role in energy transfer processes within these materials .
Cellular Effects
The compound exhibits various cellular effects, including:
- Influencing Metabolism: It affects metabolic pathways by interacting with key enzymes involved in metabolic regulation.
- Subcellular Localization: this compound localizes to specific cellular compartments, which can enhance its biological efficacy .
Research Applications
This compound has diverse applications across several fields:
- Organic Synthesis:
-
Biological Imaging:
- Its derivatives are employed in creating fluorescent dyes that are crucial for biological imaging and diagnostics.
- Material Science:
Case Studies
Case Study 1: Fluorescent Dyes Development
Researchers have utilized this compound in synthesizing BPSHY fluorophores that specifically label subcellular organelles like lysosomes and mitochondria. These dyes exhibit high quantum yields and two-photon fluorescence capabilities, demonstrating their potential for live-cell imaging applications .
Case Study 2: Organic Polymers with Ultralong RTP
A study demonstrated that grafting this compound onto polyvinyl alcohol significantly improved the RTP properties of the resulting polymers. The doped films achieved unprecedented RTP lifetimes up to 4.444 seconds, showcasing the compound's effectiveness in enhancing material performance .
Properties
IUPAC Name |
triphenylen-2-ylboronic acid | |
---|---|---|
Source | PubChem | |
URL | https://pubchem.ncbi.nlm.nih.gov | |
Description | Data deposited in or computed by PubChem | |
InChI |
InChI=1S/C18H13BO2/c20-19(21)12-9-10-17-15-7-2-1-5-13(15)14-6-3-4-8-16(14)18(17)11-12/h1-11,20-21H | |
Source | PubChem | |
URL | https://pubchem.ncbi.nlm.nih.gov | |
Description | Data deposited in or computed by PubChem | |
InChI Key |
PXFBSZZEOWJJNL-UHFFFAOYSA-N | |
Source | PubChem | |
URL | https://pubchem.ncbi.nlm.nih.gov | |
Description | Data deposited in or computed by PubChem | |
Canonical SMILES |
B(C1=CC2=C(C=C1)C3=CC=CC=C3C4=CC=CC=C42)(O)O | |
Source | PubChem | |
URL | https://pubchem.ncbi.nlm.nih.gov | |
Description | Data deposited in or computed by PubChem | |
Molecular Formula |
C18H13BO2 | |
Source | PubChem | |
URL | https://pubchem.ncbi.nlm.nih.gov | |
Description | Data deposited in or computed by PubChem | |
DSSTOX Substance ID |
DTXSID80733021 | |
Record name | Triphenylen-2-ylboronic acid | |
Source | EPA DSSTox | |
URL | https://comptox.epa.gov/dashboard/DTXSID80733021 | |
Description | DSSTox provides a high quality public chemistry resource for supporting improved predictive toxicology. | |
Molecular Weight |
272.1 g/mol | |
Source | PubChem | |
URL | https://pubchem.ncbi.nlm.nih.gov | |
Description | Data deposited in or computed by PubChem | |
CAS No. |
654664-63-8 | |
Record name | Triphenylen-2-ylboronic acid | |
Source | EPA DSSTox | |
URL | https://comptox.epa.gov/dashboard/DTXSID80733021 | |
Description | DSSTox provides a high quality public chemistry resource for supporting improved predictive toxicology. | |
Synthesis routes and methods
Procedure details
Disclaimer and Information on In-Vitro Research Products
Please be aware that all articles and product information presented on BenchChem are intended solely for informational purposes. The products available for purchase on BenchChem are specifically designed for in-vitro studies, which are conducted outside of living organisms. In-vitro studies, derived from the Latin term "in glass," involve experiments performed in controlled laboratory settings using cells or tissues. It is important to note that these products are not categorized as medicines or drugs, and they have not received approval from the FDA for the prevention, treatment, or cure of any medical condition, ailment, or disease. We must emphasize that any form of bodily introduction of these products into humans or animals is strictly prohibited by law. It is essential to adhere to these guidelines to ensure compliance with legal and ethical standards in research and experimentation.