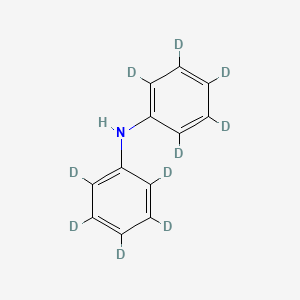
Diphenyl-D10-amine
Overview
Description
Diphenyl-D10-amine, also known as deuterated diphenylamine, is an isotope-labeled analogue of diphenylamine. It is a colorless to light yellow solid that is soluble in organic solvents such as benzene, toluene, and chloroform. The compound is primarily used in scientific research due to its unique properties imparted by the presence of deuterium atoms.
Preparation Methods
Synthetic Routes and Reaction Conditions
Diphenyl-D10-amine can be synthesized through the reaction of aniline with deuterated benzene in the presence of a catalyst. The reaction typically occurs under high temperature and pressure conditions to facilitate the incorporation of deuterium atoms into the diphenylamine structure. Another method involves the reaction of diphenylamine with deuterated sulfuric acid, which also requires high temperatures to ensure the complete exchange of hydrogen atoms with deuterium.
Industrial Production Methods
Industrial production of this compound follows similar synthetic routes but on a larger scale. The process involves the use of specialized reactors that can withstand high temperatures and pressures. The reaction mixture is continuously monitored to ensure the complete incorporation of deuterium atoms. The final product is then purified through distillation or recrystallization to obtain high-purity this compound.
Chemical Reactions Analysis
Types of Reactions
Diphenyl-D10-amine undergoes various chemical reactions, including:
Oxidation: The compound can be oxidized to form diphenylamine oxide.
Reduction: Reduction reactions can convert this compound to its corresponding amine derivatives.
Substitution: The compound can undergo substitution reactions where the hydrogen atoms are replaced by other functional groups.
Common Reagents and Conditions
Oxidation: Common oxidizing agents include potassium permanganate and hydrogen peroxide. The reactions are typically carried out in acidic or basic conditions.
Reduction: Reducing agents such as lithium aluminum hydride or sodium borohydride are used under anhydrous conditions.
Substitution: Substitution reactions often involve halogenating agents like chlorine or bromine, and the reactions are conducted under controlled temperatures to prevent unwanted side reactions.
Major Products
Oxidation: Diphenylamine oxide.
Reduction: Various amine derivatives.
Substitution: Halogenated this compound compounds.
Scientific Research Applications
Diphenyl-D10-amine is widely used in scientific research due to its unique properties. Some of its applications include:
Analytical Chemistry: Used as an internal standard in mass spectrometry and nuclear magnetic resonance (NMR) spectroscopy to study reaction mechanisms and kinetics.
Pharmaceutical Research: Employed in the synthesis of deuterated drugs to study their metabolic pathways and improve their pharmacokinetic properties.
Materials Science: Used in the development of new materials with enhanced stability and performance, particularly in high-temperature applications.
Environmental Science: Utilized in the study of environmental pollutants and their degradation pathways.
Mechanism of Action
The mechanism of action of diphenyl-D10-amine involves the interaction of its deuterium atoms with various molecular targets. The presence of deuterium atoms can alter the compound’s reactivity and stability, leading to different reaction pathways compared to its non-deuterated counterpart. The compound can act as a hydrogen donor or acceptor in various chemical reactions, influencing the overall reaction kinetics and outcomes.
Comparison with Similar Compounds
Similar Compounds
Diphenylamine: The non-deuterated analogue of diphenyl-D10-amine, commonly used as an antioxidant and stabilizer in various industrial applications.
Aniline: A simpler aromatic amine that serves as a precursor to many other compounds, including diphenylamine.
N-Phenylaniline: Another aromatic amine with similar properties to diphenylamine but with different substitution patterns.
Uniqueness
This compound is unique due to the presence of deuterium atoms, which impart distinct physical and chemical properties. These properties make it an invaluable tool in scientific research, particularly in studies involving reaction mechanisms, isotope effects, and the development of new materials and pharmaceuticals.
Biological Activity
Diphenyl-D10-amine, a deuterated derivative of diphenylamine (DPA), has garnered attention for its potential biological activities, particularly in antimicrobial and pharmaceutical applications. This article delves into the synthesis, biological properties, and research findings related to this compound.
Chemical Structure and Properties
This compound (C12H11N) features two phenyl groups attached to a nitrogen atom, with deuterium substituents enhancing its stability and providing unique analytical properties. The incorporation of deuterium can influence the compound's reactivity and biological interactions.
Synthesis of this compound Derivatives
Research has shown that various derivatives of DPA can be synthesized through reactions such as chloroacetylation and hydrazinylation. For instance, a study synthesized 2-hydrazino-N,N-diphenylacetamide derivatives, which were then evaluated for their antimicrobial properties. The synthesized compounds demonstrated significant antibacterial and antifungal activities against various strains, indicating the potential of DPA derivatives as therapeutic agents .
Antimicrobial Properties
This compound and its derivatives have been extensively studied for their antimicrobial activities. A notable study reported that specific derivatives exhibited remarkable efficacy against bacterial strains such as Bacillus pumilis, Bacillus subtilis, and Escherichia coli. The compound 2-(2-benzylidenehydrazinyl)-N,N-diphenylacetamide showed the highest antifungal activity against Rhizopus oryzae and Aspergillus niger at a concentration of 1 mg/ml, outperforming standard antibiotics like chloramphenicol .
The mechanism by which DPA derivatives exert their antimicrobial effects is believed to involve disruption of microbial cell membranes and interference with metabolic processes. The presence of electron-donating groups in the structure enhances their activity by increasing lipophilicity, which facilitates better membrane penetration .
Case Studies
-
Antimicrobial Activity Assessment :
- Study : A series of DPA derivatives were tested against multiple microbial strains.
- Findings : Compounds A1 (2-(2-benzylidenehydrazinyl)-N,N-diphenylacetamide) showed significant inhibition against fungal strains, while others demonstrated varying levels of antibacterial activity.
- : The study highlighted the potential of DPA derivatives as effective antimicrobial agents with fewer side effects compared to traditional antibiotics .
- Pharmaceutical Applications :
Safety and Toxicology
While this compound exhibits promising biological activity, safety assessments are crucial. The compound is noted to cause serious eye damage and may pose risks to fertility or fetal development according to safety data sheets. Comprehensive toxicological evaluations are essential before clinical applications can be considered .
Properties
IUPAC Name |
2,3,4,5,6-pentadeuterio-N-(2,3,4,5,6-pentadeuteriophenyl)aniline | |
---|---|---|
Source | PubChem | |
URL | https://pubchem.ncbi.nlm.nih.gov | |
Description | Data deposited in or computed by PubChem | |
InChI |
InChI=1S/C12H11N/c1-3-7-11(8-4-1)13-12-9-5-2-6-10-12/h1-10,13H/i1D,2D,3D,4D,5D,6D,7D,8D,9D,10D | |
Source | PubChem | |
URL | https://pubchem.ncbi.nlm.nih.gov | |
Description | Data deposited in or computed by PubChem | |
InChI Key |
DMBHHRLKUKUOEG-LHNTUAQVSA-N | |
Source | PubChem | |
URL | https://pubchem.ncbi.nlm.nih.gov | |
Description | Data deposited in or computed by PubChem | |
Canonical SMILES |
C1=CC=C(C=C1)NC2=CC=CC=C2 | |
Source | PubChem | |
URL | https://pubchem.ncbi.nlm.nih.gov | |
Description | Data deposited in or computed by PubChem | |
Isomeric SMILES |
[2H]C1=C(C(=C(C(=C1[2H])[2H])NC2=C(C(=C(C(=C2[2H])[2H])[2H])[2H])[2H])[2H])[2H] | |
Source | PubChem | |
URL | https://pubchem.ncbi.nlm.nih.gov | |
Description | Data deposited in or computed by PubChem | |
Molecular Formula |
C12H11N | |
Source | PubChem | |
URL | https://pubchem.ncbi.nlm.nih.gov | |
Description | Data deposited in or computed by PubChem | |
Molecular Weight |
179.28 g/mol | |
Source | PubChem | |
URL | https://pubchem.ncbi.nlm.nih.gov | |
Description | Data deposited in or computed by PubChem | |
Synthesis routes and methods I
Procedure details
Synthesis routes and methods II
Procedure details
Synthesis routes and methods III
Procedure details
Synthesis routes and methods IV
Procedure details
Synthesis routes and methods V
Procedure details
Disclaimer and Information on In-Vitro Research Products
Please be aware that all articles and product information presented on BenchChem are intended solely for informational purposes. The products available for purchase on BenchChem are specifically designed for in-vitro studies, which are conducted outside of living organisms. In-vitro studies, derived from the Latin term "in glass," involve experiments performed in controlled laboratory settings using cells or tissues. It is important to note that these products are not categorized as medicines or drugs, and they have not received approval from the FDA for the prevention, treatment, or cure of any medical condition, ailment, or disease. We must emphasize that any form of bodily introduction of these products into humans or animals is strictly prohibited by law. It is essential to adhere to these guidelines to ensure compliance with legal and ethical standards in research and experimentation.