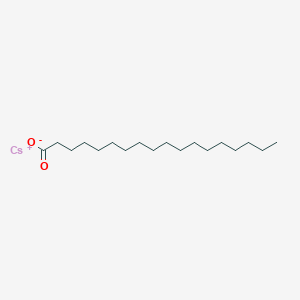
Cesium stearate
Overview
Description
Cesium stearate is a metal-organic compound, a salt formed from cesium and stearic acid. Its chemical formula is C18H35CsO2. This compound is classified as a metallic soap, which means it is a metal derivative of a fatty acid . This compound is known for its unique properties, including its solubility in hot water and its role in various industrial and scientific applications.
Preparation Methods
Synthetic Routes and Reaction Conditions: Cesium stearate can be synthesized by reacting cesium carbonate with stearic acid. The reaction typically involves heating the mixture to facilitate the formation of the salt . The general reaction is as follows: [ \text{Cs}2\text{CO}3 + 2\text{C}{18}\text{H}{36}\text{O}2 \rightarrow 2\text{C}{18}\text{H}_{35}\text{CsO}_2 + \text{H}_2\text{O} + \text{CO}_2 ]
Industrial Production Methods: In industrial settings, this compound is produced using similar methods but on a larger scale. The process involves precise control of reaction conditions, such as temperature and concentration, to ensure high yield and purity of the product. The use of high-purity reagents and advanced equipment helps in achieving consistent quality.
Chemical Reactions Analysis
Types of Reactions: Cesium stearate undergoes various chemical reactions, including:
Oxidation: this compound can be oxidized under specific conditions, leading to the formation of cesium oxide and other by-products.
Reduction: Reduction reactions involving this compound are less common but can occur under certain conditions.
Substitution: this compound can participate in substitution reactions, where the stearate group is replaced by other functional groups.
Common Reagents and Conditions:
Oxidizing Agents: Oxygen, hydrogen peroxide, and other oxidizing agents can be used for oxidation reactions.
Reducing Agents: Hydrogen gas and other reducing agents can be used for reduction reactions.
Substitution Reagents: Various organic and inorganic reagents can be used for substitution reactions.
Major Products:
Oxidation Products: Cesium oxide and other oxidized derivatives.
Reduction Products: Reduced forms of this compound.
Substitution Products: Compounds with different functional groups replacing the stearate group.
Scientific Research Applications
Cesium stearate has a wide range of applications in scientific research, including:
Biology: this compound is used in biological studies to investigate its effects on cellular processes and its potential as a therapeutic agent.
Medicine: Research is ongoing to explore the potential medical applications of this compound, including its use in drug delivery systems and as an antimicrobial agent.
Mechanism of Action
The mechanism by which cesium stearate exerts its effects involves its interaction with molecular targets and pathways. In chemical reactions, cesium ions can facilitate various processes by stabilizing transition states and intermediates . In biological systems, this compound can interact with cellular membranes and proteins, affecting their function and activity. The exact molecular targets and pathways involved are still under investigation.
Comparison with Similar Compounds
Cesium stearate can be compared with other metallic soaps, such as:
Sodium stearate: Sodium stearate is more commonly used in soaps and detergents due to its higher solubility in water.
Potassium stearate: Potassium stearate is similar to sodium stearate but has different solubility and reactivity properties.
Rubidium stearate: Rubidium stearate shares similar properties with this compound but is less commonly used due to the higher cost of rubidium.
Uniqueness: this compound is unique due to its specific properties, such as its solubility in hot water and its ability to act as a catalyst in various chemical reactions. Its applications in advanced materials and scientific research further highlight its distinctiveness.
Properties
IUPAC Name |
cesium;octadecanoate | |
---|---|---|
Source | PubChem | |
URL | https://pubchem.ncbi.nlm.nih.gov | |
Description | Data deposited in or computed by PubChem | |
InChI |
InChI=1S/C18H36O2.Cs/c1-2-3-4-5-6-7-8-9-10-11-12-13-14-15-16-17-18(19)20;/h2-17H2,1H3,(H,19,20);/q;+1/p-1 | |
Source | PubChem | |
URL | https://pubchem.ncbi.nlm.nih.gov | |
Description | Data deposited in or computed by PubChem | |
InChI Key |
WLZGEDNSZCPRCJ-UHFFFAOYSA-M | |
Source | PubChem | |
URL | https://pubchem.ncbi.nlm.nih.gov | |
Description | Data deposited in or computed by PubChem | |
Canonical SMILES |
CCCCCCCCCCCCCCCCCC(=O)[O-].[Cs+] | |
Source | PubChem | |
URL | https://pubchem.ncbi.nlm.nih.gov | |
Description | Data deposited in or computed by PubChem | |
Molecular Formula |
C18H35CsO2 | |
Source | PubChem | |
URL | https://pubchem.ncbi.nlm.nih.gov | |
Description | Data deposited in or computed by PubChem | |
Molecular Weight |
416.4 g/mol | |
Source | PubChem | |
URL | https://pubchem.ncbi.nlm.nih.gov | |
Description | Data deposited in or computed by PubChem | |
Synthesis routes and methods
Procedure details
Disclaimer and Information on In-Vitro Research Products
Please be aware that all articles and product information presented on BenchChem are intended solely for informational purposes. The products available for purchase on BenchChem are specifically designed for in-vitro studies, which are conducted outside of living organisms. In-vitro studies, derived from the Latin term "in glass," involve experiments performed in controlled laboratory settings using cells or tissues. It is important to note that these products are not categorized as medicines or drugs, and they have not received approval from the FDA for the prevention, treatment, or cure of any medical condition, ailment, or disease. We must emphasize that any form of bodily introduction of these products into humans or animals is strictly prohibited by law. It is essential to adhere to these guidelines to ensure compliance with legal and ethical standards in research and experimentation.