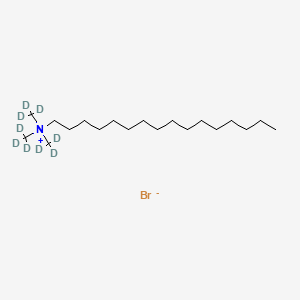
Hexadecyltrimethylammonium Bromide-d9
Overview
Description
Hexadecyltrimethylammonium Bromide-d9 (CTAB-d9) is a deuterated analogue of hexadecyltrimethylammonium bromide (CTAB), a cationic surfactant widely used in nanotechnology, colloidal science, and materials synthesis. The deuterium substitution occurs on the hexadecyl chain (C16H33 replaced by C16D33), resulting in a molecular formula of C19 ^2H33 H9 N·Br and a molecular weight of 397.65 g/mol (compared to 364.46 g/mol for non-deuterated CTAB) . This isotopic labeling is critical for analytical techniques such as nuclear magnetic resonance (NMR) and mass spectrometry, enabling precise tracking of surfactant behavior in complex systems .
CTAB-d9 retains the core physicochemical properties of CTAB, including its ability to form micelles, stabilize nanoparticles, and modify surface charges. However, its higher molecular weight and isotopic composition may slightly alter micellar thermodynamics and diffusion rates . Safety protocols for CTAB-d9 align with those of CTAB, emphasizing precautions against inhalation, skin contact, and environmental release .
Preparation Methods
Synthetic Routes and Reaction Conditions
Hexadecyltrimethylammonium Bromide-d9 can be synthesized through the quaternization of hexadecylamine with methyl bromide in the presence of deuterium oxide (D2O). The reaction typically involves heating the reactants under reflux conditions to facilitate the formation of the quaternary ammonium compound. The product is then purified through recrystallization or other suitable purification techniques .
Industrial Production Methods
In industrial settings, the production of hexadecyltrimethylammonium bromide involves large-scale quaternization reactions. The process is optimized for high yield and purity, often employing continuous flow reactors and advanced purification methods such as chromatography and crystallization .
Chemical Reactions Analysis
Types of Reactions
Hexadecyltrimethylammonium Bromide-d9 undergoes various chemical reactions, including:
Substitution Reactions: The bromide ion can be substituted with other anions in the presence of suitable reagents.
Oxidation and Reduction: The compound can participate in redox reactions, although these are less common.
Complexation: It can form complexes with metal ions, which is useful in various analytical techniques.
Common Reagents and Conditions
Substitution: Reagents such as silver nitrate (AgNO3) can be used to replace the bromide ion with a nitrate ion.
Oxidation: Strong oxidizing agents like potassium permanganate (KMnO4) can be used, although this is less typical.
Complexation: Metal salts such as copper sulfate (CuSO4) are commonly used to form complexes.
Major Products
The major products of these reactions depend on the specific reagents and conditions used. For example, substitution with silver nitrate yields hexadecyltrimethylammonium nitrate .
Scientific Research Applications
Nanotechnology Applications
Synthesis of Nanostructures
Hexadecyltrimethylammonium bromide-d9 plays a crucial role in the synthesis of metal nanostructures, particularly gold and silver nanorods. The surfactant stabilizes the nanostructures during the synthesis process, influencing their size and shape.
- Gold Nanorods : The surfactant assists in the formation of gold nanorods with specific surface plasmon resonance properties, which are essential for applications in photothermal therapy and imaging .
Nanostructure | Surfactant Role | Application |
---|---|---|
Gold Nanorods | Stabilization | Photothermal therapy, imaging |
Silver Nanostructures | Shape control | Catalysis, sensing |
Biochemical Applications
Homogenization in Biochemical Assays
this compound is employed as a component in buffer solutions for tissue homogenization. This process is critical for determining enzyme activities, such as myeloperoxidase activity in biological samples.
- Enzyme Activity Measurement : The surfactant enhances the extraction efficiency of enzymes from tissues, facilitating accurate biochemical assays .
Application | Description |
---|---|
Tissue Homogenization | Enhances enzyme extraction for activity assays |
Enzyme Activity Assays | Used in buffers to measure specific enzyme functions |
Microemulsion Systems
Catalysis in Microemulsions
this compound is also utilized in microemulsion systems where it acts as a catalyst for various chemical reactions. For instance, studies have shown its effectiveness in catalyzing the degradation of beta-lactam antibiotics like cephaclor.
- Catalytic Properties : The surfactant's ability to form microemulsions significantly affects reaction rates and mechanisms, providing insights into drug stability and degradation pathways .
Reaction Type | Surfactant Role | Impact on Reaction |
---|---|---|
Beta-lactam Degradation | Catalyst in microemulsion | Increased reaction rate |
Organic Synthesis | Reaction medium | Improved product yield |
Material Science
Liquid Crystal Alignment
Recent studies have explored the use of this compound in aligning liquid crystals for display technologies. Its surface-active properties facilitate the alignment of liquid crystal molecules under varying conditions.
- Display Technology : This application is particularly relevant for improving the performance of liquid crystal displays (LCDs) by optimizing the alignment of liquid crystal layers .
Material Application | Functionality |
---|---|
Liquid Crystal Displays | Enhances alignment properties |
Layered ITO Cells | Improves performance under varied conditions |
Case Studies
Several case studies highlight the effectiveness of this compound across different applications:
- Gold Nanorod Synthesis Study : A study demonstrated that varying concentrations of this compound could control the aspect ratio of gold nanorods, influencing their optical properties significantly .
- Microemulsion Catalysis Research : Research indicated that microemulsions containing this compound exhibited enhanced catalytic activity for antibiotic degradation compared to traditional methods, suggesting its potential in pharmaceutical applications .
Mechanism of Action
Hexadecyltrimethylammonium Bromide-d9 exerts its effects primarily through its surfactant properties. It reduces surface tension and forms micelles, which can encapsulate hydrophobic molecules. This property is particularly useful in DNA extraction, where it helps to lyse cells and solubilize membrane lipids. The compound also binds to polysaccharides, aiding in their removal during the extraction process .
Comparison with Similar Compounds
Structural and Functional Analogues
Table 1: Key Properties of CTAB-d9 and Related Surfactants
Table 2: Surfactant-Dependent Morphology of Ag−Pd Nanoparticles
Surfactant | Counterion | Nanoparticle Shape | Key Parameter Influenced |
---|---|---|---|
CTAB | Br⁻ | Nanocubes | Crystal facet stabilization |
CTAC + NaOL | Cl⁻ | Nanodendrites | Branching via oleate coordination |
CTAB’s bromide ion promotes the formation of Ag−Pd nanocubes by stabilizing (100) crystal facets, while CTAC (with chloride) combined with sodium oleate induces dendritic growth due to altered ion coordination . CTAB-d9 is expected to behave similarly to CTAB in such systems but may enable isotopic labeling for mechanistic studies.
Colloidal Stability in Ferrofluids
Table 3: Comparison of CTAB and DBSA in Barium Hexaferrite (BHF) Ferrofluids
Parameter | CTAB-Coated BHF | DBSA-Coated BHF |
---|---|---|
Zeta Potential (mV) | -45 to -55 | -30 to -40 |
Surfactant Adsorption | Strong (resists dilution) | Weak (desorbs readily) |
Carrier Fluid Compatibility | Ethylene glycol (high stability) | Butanol (volatile) |
CTAB’s cationic head group binds strongly to negatively charged BHF nanoparticles, yielding higher zeta potentials and better colloidal stability in ethylene glycol compared to anionic DBSA . CTAB-d9 would exhibit similar performance, with deuterium substitution minimally affecting interfacial interactions.
Thermodynamic Behavior in Micelle Formation
The micellization thermodynamics of CTAB in aqueous urea solutions were studied via isothermal titration calorimetry. Key parameters include:
- ΔG: -28.5 kJ/mol (micelle formation is spontaneous)
- ΔH: +2.1 kJ/mol (endothermic process)
- ΔS: +102 J/mol·K (entropy-driven)
Comparatively, SDS (anionic) and DBSA (bulky aromatic tail) exhibit lower entropy gains due to differences in head-group hydration and tail packing. CTAC’s micellization is less entropy-driven owing to smaller chloride counterions .
Electrical Double Layer and Surface Charge Modulation
CTAB’s ability to form self-assembled structures with well-defined electrical double layers (EDLs) has been extensively characterized . In contrast to tetramethylammonium bromide (TMABr), CTAB’s long alkyl chain enhances EDL thickness and surface charge density, making it superior for stabilizing charged nanoparticles . Deuterated CTAB-d9 would maintain these properties, with isotopic effects negligible in most applications.
Biological Activity
Hexadecyltrimethylammonium Bromide-d9 (HTAB-d9), a deuterated form of the well-known cationic surfactant hexadecyltrimethylammonium bromide (CTAB), has garnered interest in various biological and chemical applications due to its unique properties. This article aims to provide a comprehensive overview of the biological activity of HTAB-d9, including its mechanisms, applications, and relevant case studies.
- Chemical Formula : C19H42BrN (with deuterium substitution)
- Molecular Weight : 364.46 g/mol
- CAS Number : 95217-14-4
- Structure : HTAB-d9 consists of a long hydrophobic alkyl chain (hexadecane) and a positively charged quaternary ammonium group, which imparts surfactant properties.
Mechanisms of Biological Activity
HTAB-d9 exhibits biological activity primarily through its surfactant properties, which affect cell membranes and influence various biochemical processes. The following mechanisms are notable:
- Membrane Disruption : HTAB-d9 can integrate into lipid bilayers, altering membrane fluidity and permeability. This property is crucial for its application in drug delivery systems where enhanced membrane permeability can facilitate the uptake of therapeutic agents into cells .
- Antimicrobial Activity : Similar to its non-deuterated counterpart, HTAB-d9 has demonstrated antimicrobial properties against a range of bacteria and fungi. Its cationic nature allows it to interact with negatively charged microbial membranes, leading to cell lysis .
- Cellular Uptake Enhancement : Studies have shown that HTAB-d9 can enhance the cellular uptake of nanoparticles and drugs, making it a valuable additive in formulations designed for targeted delivery .
Antimicrobial Efficacy
A study investigating the antimicrobial effects of CTAB derivatives, including HTAB-d9, reported significant inhibition of Gram-positive bacteria and fungi. The study utilized various concentrations of the surfactant and assessed its impact on bacterial growth rates .
Surfactant Concentration (mg/mL) | Zone of Inhibition (mm) | Bacterial Strain |
---|---|---|
0.5 | 15 | Staphylococcus aureus |
1.0 | 22 | Escherichia coli |
2.0 | 30 | Candida albicans |
Drug Delivery Applications
In drug delivery studies, HTAB-d9 was incorporated into liposomal formulations to enhance the delivery of chemotherapeutic agents. The results indicated improved drug encapsulation efficiency and enhanced cytotoxicity against cancer cells compared to control formulations without HTAB-d9 .
Cytotoxicity Assessment
While HTAB-d9 shows promise in enhancing drug delivery, its cytotoxic effects were also evaluated. A study examining the effects on human basophil cells indicated that higher concentrations led to increased apoptosis rates . This highlights the need for careful optimization in therapeutic applications.
Summary of Biological Activities
HTAB-d9 demonstrates significant biological activities that can be leveraged in various fields, including:
- Antimicrobial formulations : Effective against a broad spectrum of pathogens.
- Drug delivery systems : Enhances cellular uptake and bioavailability.
- Biochemical research : Useful as a tool for studying membrane dynamics and interactions.
Q & A
Q. Basic: How can researchers determine the critical micelle concentration (CMC) and aggregation number of HTAB in aqueous solutions with varying counterions?
Methodological Answer:
The CMC and aggregation number of HTAB can be determined using electrical conductivity measurements and the pseudo-phase separation model . Conductivity data is plotted against surfactant concentration, with the CMC identified as the inflection point. Aggregation numbers are derived from fluorescence quenching or static light scattering.
-
Key Factor: Counterion valency significantly impacts micellization. For example, disodium hydrogen phosphate (divalent anion) reduces HTAB's CMC more effectively than monovalent salts like NaCl due to stronger electrostatic screening .
-
Sodium Salt Anion Valency CMC Trend Aggregation Number Trend Disodium hydrogen phosphate 2 Decreases Increases Sodium chloride 1 Moderate Moderate
Q. Basic: What experimental approaches are used to study HTAB’s role in nanomaterial morphology control?
Methodological Answer:
HTAB acts as a structure-directing agent in nanomaterial synthesis. Researchers use:
- TEM/SEM Imaging: To analyze nanoparticle size and shape (e.g., gold helicoids or silica aerogels) .
- Concentration Gradients: Varying HTAB concentrations (e.g., 0.1–1.0 mM) to tune pore size in mesoporous zeolites .
- Co-surfactant Systems: Combining HTAB with ascorbic acid or glutathione to stabilize chiral nanostructures .
Q. Advanced: How can contradictory data on HTAB’s micellization behavior with different salts be resolved?
Methodological Answer:
Contradictions arise from anion-specific effects. Systematic analysis involves:
Standard Gibbs Free Energy (ΔG°mic): Calculate using the pseudo-phase separation model to compare micelle stability across salts .
Ion-Specific Trends: Higher-valency anions (e.g., PO4<sup>3-</sup>) reduce CMC by enhancing counterion binding.
Dynamic Light Scattering (DLS): Measure hydrodynamic radii to correlate aggregation numbers with salt type.
Q. Advanced: What methodologies elucidate HTAB’s role in chiral nanostructure synthesis without chiral ligands?
Methodological Answer:
HTAB’s surface-active properties enable chirality via:
- Morphological Control: Adjusting HTAB concentration to induce asymmetric growth in gold helicoids (e.g., 432 helicoid III nanoparticles) .
- Kinetic Studies: Monitoring time-dependent chiral evolution using circular dichroism (CD) spectroscopy.
- Competitive Adsorption: Replacing CTAB with CTAC (chloride analog) to isolate bromide’s role in Au{100} facet stabilization .
Q. Advanced: How is HTAB optimized for interfacial tension studies in enhanced oil recovery (EOR)?
Methodological Answer:
HTAB’s amphiphilic nature reduces oil-water interfacial tension (IFT). Key methodologies include:
- IFT Measurements: Using pendant drop tensiometry with ZnO nanoparticles to study synergistic effects .
- Adsorption Isotherms: Applying Langmuir/Freundlich models to HTAB-modified adsorbents (e.g., alum sludge) .
- Salt Tolerance Tests: Evaluating HTAB performance in high-salinity reservoirs (e.g., 5–20% NaCl) .
Q. Advanced: What protocols mitigate HTAB’s environmental impact in lab settings?
Methodological Answer:
HTAB’s aquatic toxicity (EC50 < 1 mg/L for algae) necessitates:
- Waste Neutralization: Acidic hydrolysis (pH < 3) to decompose HTAB before disposal .
- Adsorption Treatments: Using activated carbon or modified clays to remove HTAB from lab wastewater .
- Alternative Surfactants: Testing biodegradable analogs (e.g., sugar-based surfactants) for non-critical applications.
Note on Isotopic Effects (HTAB-d9):
While the provided evidence focuses on HTAB (non-deuterated), methodologies for HTAB-d9 would require adjustments for isotopic labeling, such as NMR spectroscopy to track deuterium in self-assembly kinetics or metabolic studies.
Properties
IUPAC Name |
hexadecyl-tris(trideuteriomethyl)azanium;bromide | |
---|---|---|
Source | PubChem | |
URL | https://pubchem.ncbi.nlm.nih.gov | |
Description | Data deposited in or computed by PubChem | |
InChI |
InChI=1S/C19H42N.BrH/c1-5-6-7-8-9-10-11-12-13-14-15-16-17-18-19-20(2,3)4;/h5-19H2,1-4H3;1H/q+1;/p-1/i2D3,3D3,4D3; | |
Source | PubChem | |
URL | https://pubchem.ncbi.nlm.nih.gov | |
Description | Data deposited in or computed by PubChem | |
InChI Key |
LZZYPRNAOMGNLH-WWMMTMLWSA-M | |
Source | PubChem | |
URL | https://pubchem.ncbi.nlm.nih.gov | |
Description | Data deposited in or computed by PubChem | |
Canonical SMILES |
CCCCCCCCCCCCCCCC[N+](C)(C)C.[Br-] | |
Source | PubChem | |
URL | https://pubchem.ncbi.nlm.nih.gov | |
Description | Data deposited in or computed by PubChem | |
Isomeric SMILES |
[2H]C([2H])([2H])[N+](CCCCCCCCCCCCCCCC)(C([2H])([2H])[2H])C([2H])([2H])[2H].[Br-] | |
Source | PubChem | |
URL | https://pubchem.ncbi.nlm.nih.gov | |
Description | Data deposited in or computed by PubChem | |
Molecular Formula |
C19H42BrN | |
Source | PubChem | |
URL | https://pubchem.ncbi.nlm.nih.gov | |
Description | Data deposited in or computed by PubChem | |
Molecular Weight |
373.5 g/mol | |
Source | PubChem | |
URL | https://pubchem.ncbi.nlm.nih.gov | |
Description | Data deposited in or computed by PubChem | |
Synthesis routes and methods
Procedure details
Disclaimer and Information on In-Vitro Research Products
Please be aware that all articles and product information presented on BenchChem are intended solely for informational purposes. The products available for purchase on BenchChem are specifically designed for in-vitro studies, which are conducted outside of living organisms. In-vitro studies, derived from the Latin term "in glass," involve experiments performed in controlled laboratory settings using cells or tissues. It is important to note that these products are not categorized as medicines or drugs, and they have not received approval from the FDA for the prevention, treatment, or cure of any medical condition, ailment, or disease. We must emphasize that any form of bodily introduction of these products into humans or animals is strictly prohibited by law. It is essential to adhere to these guidelines to ensure compliance with legal and ethical standards in research and experimentation.