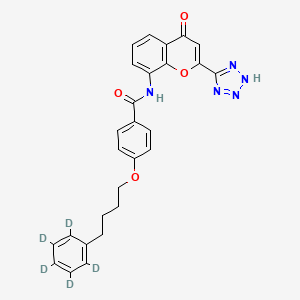
Pranlukast-d5
Overview
Description
Pranlukast-d5 is a deuterated form of Pranlukast, a cysteinyl leukotriene receptor-1 antagonist. It is primarily used in scientific research to study the pharmacokinetics and metabolic pathways of Pranlukast. The deuterium atoms in this compound replace hydrogen atoms, which can help in tracing the compound in biological systems without altering its pharmacological properties.
Mechanism of Action
Target of Action
Pranlukast-d5 primarily targets the Cysteinyl Leukotriene Receptor 1 (CysLT1) . This receptor plays a crucial role in mediating bronchospasm, which is a significant symptom in conditions like asthma .
Mode of Action
This compound acts as an antagonist to the CysLT1 receptor . It selectively binds to this receptor and inhibits the actions of Leukotriene D4 (LTD4), a potent mediator of inflammation . By doing so, it prevents airway edema, smooth muscle contraction, and the secretion of thick, viscous mucus .
Biochemical Pathways
The primary biochemical pathway affected by this compound is the leukotriene pathway . By antagonizing the CysLT1 receptor, this compound inhibits the inflammatory response triggered by leukotrienes, thereby alleviating symptoms of asthma and allergic rhinitis .
Pharmacokinetics
It is known that the compound undergoes hepatic metabolism
Result of Action
The antagonistic action of this compound on the CysLT1 receptor results in the prevention of airway edema, smooth muscle contraction, and enhanced secretion of thick, viscous mucus . This leads to relief from symptoms of conditions like asthma and allergic rhinitis .
Action Environment
The efficacy and stability of this compound can be influenced by various environmental factors. For instance, the time of dosing appears to influence the pharmacokinetics of Pranlukast . .
Biochemical Analysis
Biochemical Properties
Pranlukast-d5 interacts with cysteinyl leukotriene receptor-1, a protein found in the human airway . It selectively antagonizes leukotriene D4 (LTD4) at this receptor . This interaction prevents airway edema, smooth muscle contraction, and enhanced secretion of thick, viscous mucus .
Cellular Effects
This compound has significant effects on various types of cells and cellular processes. It influences cell function by preventing bronchospasm caused by an allergic reaction to allergens . This is particularly beneficial for asthmatics .
Molecular Mechanism
The molecular mechanism of this compound involves its selective antagonism of leukotriene D4 (LTD4) at the cysteinyl leukotriene receptor, CysLT1, in the human airway . This antagonism inhibits the actions of LTD4 at the CysLT1 receptor, preventing airway edema, smooth muscle contraction, and enhanced secretion of thick, viscous mucus .
Dosage Effects in Animal Models
It is known that Pranlukast has shown a range of anti-inflammatory effects on airways in animal models .
Metabolic Pathways
This compound is involved in the arginine biosynthesis pathway of Mycobacterium tuberculosis . It allosterically inhibits ornithine acetyltransferase (MtArgJ), a crucial enzyme in this pathway .
Transport and Distribution
It is known that Pranlukast, the parent compound, is metabolized hepatically, mainly by CYP3A4 .
Subcellular Localization
Given its biochemical properties and interactions, it is likely to be found in areas where the cysteinyl leukotriene receptor-1 is present, such as the human airway .
Preparation Methods
Synthetic Routes and Reaction Conditions: The synthesis of Pranlukast-d5 involves multiple steps, starting with the preparation of deuterated intermediates. The key steps include:
Synthesis of Deuterated Benzopyran Intermediate: This involves the nitration of a deuterated benzopyran compound, followed by reduction to form the corresponding amine.
Formation of Deuterated Tetrazole: The amine is then reacted with sodium azide and triethyl orthoformate to form the tetrazole ring.
Coupling Reaction: The deuterated benzopyran-tetrazole intermediate is coupled with a deuterated phenylbutoxybenzamide under specific conditions to form this compound.
Industrial Production Methods: Industrial production of this compound follows similar synthetic routes but on a larger scale. The process involves:
Bulk Synthesis of Deuterated Intermediates: Using deuterated reagents and solvents to ensure high deuterium incorporation.
Optimization of Reaction Conditions: Ensuring high yield and purity through controlled reaction conditions such as temperature, pressure, and pH.
Purification: Using techniques like crystallization, chromatography, and recrystallization to obtain high-purity this compound.
Chemical Reactions Analysis
Types of Reactions: Pranlukast-d5 undergoes various chemical reactions, including:
Oxidation: this compound can be oxidized using reagents like hydrogen peroxide or potassium permanganate to form corresponding oxides.
Reduction: Reduction reactions using agents like lithium aluminum hydride can convert this compound to its reduced forms.
Substitution: Nucleophilic substitution reactions can occur at specific positions in the molecule, leading to the formation of substituted derivatives.
Common Reagents and Conditions:
Oxidation: Hydrogen peroxide, potassium permanganate, and other oxidizing agents under acidic or basic conditions.
Reduction: Lithium aluminum hydride, sodium borohydride, and other reducing agents under anhydrous conditions.
Substitution: Nucleophiles like halides, amines, and thiols under appropriate solvent and temperature conditions.
Major Products: The major products formed from these reactions include oxidized, reduced, and substituted derivatives of this compound, which can be further analyzed for their pharmacological properties.
Scientific Research Applications
Pranlukast-d5 has several scientific research applications, including:
Pharmacokinetic Studies: Used to trace the metabolic pathways and distribution of Pranlukast in biological systems.
Metabolic Studies: Helps in identifying the metabolites of Pranlukast and understanding its biotransformation.
Drug Interaction Studies: Used to study the interactions of Pranlukast with other drugs and their impact on its pharmacokinetics.
Analytical Chemistry: Employed as an internal standard in mass spectrometry and other analytical techniques to quantify Pranlukast in biological samples.
Comparison with Similar Compounds
Pranlukast-d5 is compared with other cysteinyl leukotriene receptor antagonists such as:
Montelukast: Another leukotriene receptor antagonist with a similar mechanism of action but different pharmacokinetic properties.
Zafirlukast: Similar to Pranlukast but with different binding affinities and metabolic pathways.
Ibudilast: Although not a leukotriene receptor antagonist, it has anti-inflammatory properties and is used in asthma treatment.
Uniqueness of this compound: this compound’s uniqueness lies in its deuterium incorporation, which allows for detailed pharmacokinetic and metabolic studies without altering the compound’s pharmacological effects. This makes it a valuable tool in drug development and research.
Properties
IUPAC Name |
N-[4-oxo-2-(2H-tetrazol-5-yl)chromen-8-yl]-4-[4-(2,3,4,5,6-pentadeuteriophenyl)butoxy]benzamide | |
---|---|---|
Source | PubChem | |
URL | https://pubchem.ncbi.nlm.nih.gov | |
Description | Data deposited in or computed by PubChem | |
InChI |
InChI=1S/C27H23N5O4/c33-23-17-24(26-29-31-32-30-26)36-25-21(23)10-6-11-22(25)28-27(34)19-12-14-20(15-13-19)35-16-5-4-9-18-7-2-1-3-8-18/h1-3,6-8,10-15,17H,4-5,9,16H2,(H,28,34)(H,29,30,31,32)/i1D,2D,3D,7D,8D | |
Source | PubChem | |
URL | https://pubchem.ncbi.nlm.nih.gov | |
Description | Data deposited in or computed by PubChem | |
InChI Key |
NBQKINXMPLXUET-RCQSQLKUSA-N | |
Source | PubChem | |
URL | https://pubchem.ncbi.nlm.nih.gov | |
Description | Data deposited in or computed by PubChem | |
Canonical SMILES |
C1=CC=C(C=C1)CCCCOC2=CC=C(C=C2)C(=O)NC3=CC=CC4=C3OC(=CC4=O)C5=NNN=N5 | |
Source | PubChem | |
URL | https://pubchem.ncbi.nlm.nih.gov | |
Description | Data deposited in or computed by PubChem | |
Isomeric SMILES |
[2H]C1=C(C(=C(C(=C1[2H])[2H])CCCCOC2=CC=C(C=C2)C(=O)NC3=CC=CC4=C3OC(=CC4=O)C5=NNN=N5)[2H])[2H] | |
Source | PubChem | |
URL | https://pubchem.ncbi.nlm.nih.gov | |
Description | Data deposited in or computed by PubChem | |
Molecular Formula |
C27H23N5O4 | |
Source | PubChem | |
URL | https://pubchem.ncbi.nlm.nih.gov | |
Description | Data deposited in or computed by PubChem | |
Molecular Weight |
486.5 g/mol | |
Source | PubChem | |
URL | https://pubchem.ncbi.nlm.nih.gov | |
Description | Data deposited in or computed by PubChem | |
Synthesis routes and methods
Procedure details
Disclaimer and Information on In-Vitro Research Products
Please be aware that all articles and product information presented on BenchChem are intended solely for informational purposes. The products available for purchase on BenchChem are specifically designed for in-vitro studies, which are conducted outside of living organisms. In-vitro studies, derived from the Latin term "in glass," involve experiments performed in controlled laboratory settings using cells or tissues. It is important to note that these products are not categorized as medicines or drugs, and they have not received approval from the FDA for the prevention, treatment, or cure of any medical condition, ailment, or disease. We must emphasize that any form of bodily introduction of these products into humans or animals is strictly prohibited by law. It is essential to adhere to these guidelines to ensure compliance with legal and ethical standards in research and experimentation.