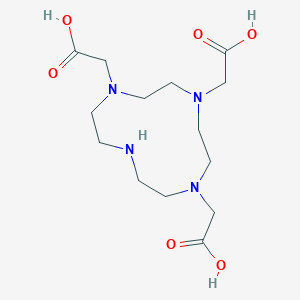
1,4,7,10-Tetraazacyclododecane-1,4,7-triacetic acid
Overview
Description
1,4,7-Tris(carboxymethyl)-1,4,7,10-tetraazacyclododecane is a macrocyclic compound known for its ability to chelate metal ions. This compound is particularly significant in the field of coordination chemistry due to its high affinity for lanthanides and transition metals. It forms thermodynamically stable and kinetically inert complexes, making it useful in various scientific and industrial applications .
Mechanism of Action
Target of Action
It is known to be an impurity of gadobutrol , a nonionic, paramagnetic contrast agent used in magnetic resonance imaging (MRI) . Gadobutrol primarily targets water molecules in the body to enhance the contrast in MRI scans .
Mode of Action
As an impurity of gadobutrol, it may share similar properties . Gadobutrol works by altering the magnetic properties of water molecules in the body, which enhances the contrast between different tissues in MRI scans .
Pharmacokinetics
As an impurity of Gadobutrol, it may share similar pharmacokinetic properties .
Result of Action
As an impurity of Gadobutrol, it may share similar effects, such as enhancing the contrast in MRI scans .
Biochemical Analysis
Cellular Effects
It is known that it can influence cell function, potentially impacting cell signaling pathways, gene expression, and cellular metabolism
Molecular Mechanism
It is known to bind to certain biomolecules, potentially influencing enzyme activity and gene expression
Temporal Effects in Laboratory Settings
Similarly, any long-term effects on cellular function observed in in vitro or in vivo studies have not been well studied .
Preparation Methods
Synthetic Routes and Reaction Conditions
The synthesis of 1,4,7-Tris(carboxymethyl)-1,4,7,10-tetraazacyclododecane typically involves the alkylation of 1,4,7,10-tetraazacyclododecane with bromoacetic acid under basic conditions. The reaction is carried out in a solvent such as acetonitrile, and the product is purified through recrystallization or chromatography .
Industrial Production Methods
Industrial production of this compound follows similar synthetic routes but on a larger scale. The process involves the use of automated reactors and continuous flow systems to ensure high yield and purity. The reaction conditions are optimized to minimize by-products and maximize efficiency .
Chemical Reactions Analysis
Types of Reactions
1,4,7-Tris(carboxymethyl)-1,4,7,10-tetraazacyclododecane undergoes various chemical reactions, including:
Chelation: Forms stable complexes with metal ions such as gadolinium, copper, and zinc.
Substitution: Can undergo substitution reactions where the carboxymethyl groups are replaced with other functional groups.
Common Reagents and Conditions
Chelation: Typically involves metal salts like gadolinium chloride in aqueous or methanolic solutions.
Substitution: Uses reagents such as alkyl halides or acyl chlorides under basic or acidic conditions.
Major Products
Scientific Research Applications
1,4,7-Tris(carboxymethyl)-1,4,7,10-tetraazacyclododecane has a wide range of applications in scientific research:
Comparison with Similar Compounds
Similar Compounds
1,4,7,10-Tetraazacyclododecane-1,4,7,10-tetraacetic acid (DOTA): Another macrocyclic compound with similar chelating properties.
1,4,7-Tris(aminocarbonylmethyl)-1,4,7,10-tetraazacyclododecane: A derivative with different functional groups, offering varied reactivity and applications.
Uniqueness
1,4,7-Tris(carboxymethyl)-1,4,7,10-tetraazacyclododecane is unique due to its high affinity for lanthanides and its ability to form kinetically inert complexes. This makes it particularly useful in applications requiring stable and long-lasting metal complexes, such as in medical imaging and industrial catalysis .
Biological Activity
1,4,7,10-Tetraazacyclododecane-1,4,7-triacetic acid (DOTA) is a versatile chelating agent widely used in medical imaging and targeted radionuclide therapy. This compound's biological activity is primarily attributed to its ability to form stable complexes with various metal ions, which enhances its utility in diagnostic and therapeutic applications.
DOTA is a cyclic tetraamine with four nitrogen atoms and three carboxylic acid groups. Its molecular formula is , and it has a molecular weight of approximately 288.34 g/mol. The structure allows for the coordination of metal ions through the nitrogen atoms and carboxylate groups, facilitating the formation of stable chelates.
DOTA's biological activity can be understood through its interaction with metal ions:
- Chelation : DOTA effectively binds to metal ions such as gadolinium (Gd), lutetium (Lu), and copper (Cu), forming stable complexes that can be utilized in imaging and therapy.
- Targeting Tumors : When conjugated to biomolecules like peptides or antibodies, DOTA-labeled compounds can target specific tumor cells, enhancing the delivery of therapeutic agents directly to cancerous tissues.
Imaging
DOTA is extensively used in radiopharmaceuticals for imaging applications:
- MRI Contrast Agents : DOTA-Gd complexes are employed as contrast agents in magnetic resonance imaging (MRI) due to their paramagnetic properties, which enhance image contrast.
- Positron Emission Tomography (PET) : DOTA-labeled compounds are used in PET imaging for tracking biological processes and diagnosing diseases such as cancer.
Therapeutic Applications
The therapeutic potential of DOTA is significant:
- Radionuclide Therapy : DOTA can be conjugated with radioactive isotopes like for targeted radionuclide therapy (TRNT). This approach allows for localized radiation delivery to tumors while minimizing damage to surrounding healthy tissues.
- Clinical Trials : Several clinical studies have demonstrated the efficacy of DOTA-conjugated radiopharmaceuticals in treating various cancers. For instance, studies involving have shown promising results in neuroendocrine tumors.
Case Study 1: Alzheimer's Disease Imaging
A study investigated the use of DOTA-based complexes for imaging amyloid-beta plaques in Alzheimer’s disease models. The research demonstrated that DOTA-labeled compounds showed high brain uptake in transgenic mice models compared to wild-type mice, indicating potential for early diagnosis of Alzheimer's disease through non-invasive imaging techniques .
Case Study 2: Targeted Radionuclide Therapy
In another study focusing on targeted radionuclide therapy using , patients with metastatic neuroendocrine tumors exhibited significant tumor reduction after treatment. The study highlighted the effectiveness of DOTA as a chelator that stabilizes radioactive isotopes for therapeutic use while maintaining low toxicity levels .
Comparative Analysis of DOTA Complexes
The following table summarizes the biological activity and applications of various DOTA-metal complexes:
Properties
IUPAC Name |
2-[4,7-bis(carboxymethyl)-1,4,7,10-tetrazacyclododec-1-yl]acetic acid | |
---|---|---|
Source | PubChem | |
URL | https://pubchem.ncbi.nlm.nih.gov | |
Description | Data deposited in or computed by PubChem | |
InChI |
InChI=1S/C14H26N4O6/c19-12(20)9-16-3-1-15-2-4-17(10-13(21)22)6-8-18(7-5-16)11-14(23)24/h15H,1-11H2,(H,19,20)(H,21,22)(H,23,24) | |
Source | PubChem | |
URL | https://pubchem.ncbi.nlm.nih.gov | |
Description | Data deposited in or computed by PubChem | |
InChI Key |
HHLZCENAOIROSL-UHFFFAOYSA-N | |
Source | PubChem | |
URL | https://pubchem.ncbi.nlm.nih.gov | |
Description | Data deposited in or computed by PubChem | |
Canonical SMILES |
C1CN(CCN(CCN(CCN1)CC(=O)O)CC(=O)O)CC(=O)O | |
Source | PubChem | |
URL | https://pubchem.ncbi.nlm.nih.gov | |
Description | Data deposited in or computed by PubChem | |
Molecular Formula |
C14H26N4O6 | |
Source | PubChem | |
URL | https://pubchem.ncbi.nlm.nih.gov | |
Description | Data deposited in or computed by PubChem | |
DSSTOX Substance ID |
DTXSID60276042 | |
Record name | 1,4,7-tris(carboxymethyl)-1,4,7,10-tetraazacyclododecane | |
Source | EPA DSSTox | |
URL | https://comptox.epa.gov/dashboard/DTXSID60276042 | |
Description | DSSTox provides a high quality public chemistry resource for supporting improved predictive toxicology. | |
Molecular Weight |
346.38 g/mol | |
Source | PubChem | |
URL | https://pubchem.ncbi.nlm.nih.gov | |
Description | Data deposited in or computed by PubChem | |
CAS No. |
114873-37-9 | |
Record name | 1,4,7-Tris(carboxymethyl)-1,4,7,10-tetraazacyclododecane | |
Source | ChemIDplus | |
URL | https://pubchem.ncbi.nlm.nih.gov/substance/?source=chemidplus&sourceid=0114873379 | |
Description | ChemIDplus is a free, web search system that provides access to the structure and nomenclature authority files used for the identification of chemical substances cited in National Library of Medicine (NLM) databases, including the TOXNET system. | |
Record name | 1,4,7-tris(carboxymethyl)-1,4,7,10-tetraazacyclododecane | |
Source | EPA DSSTox | |
URL | https://comptox.epa.gov/dashboard/DTXSID60276042 | |
Description | DSSTox provides a high quality public chemistry resource for supporting improved predictive toxicology. | |
Record name | 1,4,7,10-Tetraazacyclododecane-1,4,7-triacetic acid | |
Source | FDA Global Substance Registration System (GSRS) | |
URL | https://gsrs.ncats.nih.gov/ginas/app/beta/substances/A6DV6KJV7Z | |
Description | The FDA Global Substance Registration System (GSRS) enables the efficient and accurate exchange of information on what substances are in regulated products. Instead of relying on names, which vary across regulatory domains, countries, and regions, the GSRS knowledge base makes it possible for substances to be defined by standardized, scientific descriptions. | |
Explanation | Unless otherwise noted, the contents of the FDA website (www.fda.gov), both text and graphics, are not copyrighted. They are in the public domain and may be republished, reprinted and otherwise used freely by anyone without the need to obtain permission from FDA. Credit to the U.S. Food and Drug Administration as the source is appreciated but not required. | |
Synthesis routes and methods I
Procedure details
Synthesis routes and methods II
Procedure details
Synthesis routes and methods III
Procedure details
Retrosynthesis Analysis
AI-Powered Synthesis Planning: Our tool employs the Template_relevance Pistachio, Template_relevance Bkms_metabolic, Template_relevance Pistachio_ringbreaker, Template_relevance Reaxys, Template_relevance Reaxys_biocatalysis model, leveraging a vast database of chemical reactions to predict feasible synthetic routes.
One-Step Synthesis Focus: Specifically designed for one-step synthesis, it provides concise and direct routes for your target compounds, streamlining the synthesis process.
Accurate Predictions: Utilizing the extensive PISTACHIO, BKMS_METABOLIC, PISTACHIO_RINGBREAKER, REAXYS, REAXYS_BIOCATALYSIS database, our tool offers high-accuracy predictions, reflecting the latest in chemical research and data.
Strategy Settings
Precursor scoring | Relevance Heuristic |
---|---|
Min. plausibility | 0.01 |
Model | Template_relevance |
Template Set | Pistachio/Bkms_metabolic/Pistachio_ringbreaker/Reaxys/Reaxys_biocatalysis |
Top-N result to add to graph | 6 |
Feasible Synthetic Routes
Disclaimer and Information on In-Vitro Research Products
Please be aware that all articles and product information presented on BenchChem are intended solely for informational purposes. The products available for purchase on BenchChem are specifically designed for in-vitro studies, which are conducted outside of living organisms. In-vitro studies, derived from the Latin term "in glass," involve experiments performed in controlled laboratory settings using cells or tissues. It is important to note that these products are not categorized as medicines or drugs, and they have not received approval from the FDA for the prevention, treatment, or cure of any medical condition, ailment, or disease. We must emphasize that any form of bodily introduction of these products into humans or animals is strictly prohibited by law. It is essential to adhere to these guidelines to ensure compliance with legal and ethical standards in research and experimentation.