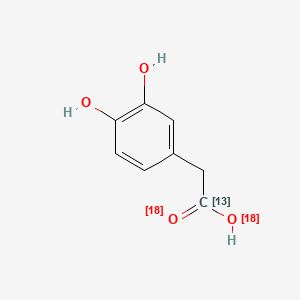
2-(3,4-dihydroxyphenyl)acetic acid
Overview
Description
Preparation Methods
Synthetic Routes and Reaction Conditions
One common method for synthesizing 2-(3,4-dihydroxyphenyl)acetic acid involves the aerobic biotransformation of 4-hydroxyphenylacetic acid using whole cell cultures of Arthrobacter protophormiae. This method yields the product with a 52% efficiency . Another approach involves the biosynthesis using metabolic engineering techniques, where 4-hydroxyphenylacetic acid is converted to this compound using high-efficiency expression of hydroxylase enzymes .
Industrial Production Methods
Industrial production of this compound can be achieved through biotechnological processes involving genetically engineered microorganisms. These microorganisms are designed to express specific enzymes that facilitate the conversion of precursor compounds into the desired product .
Chemical Reactions Analysis
Types of Reactions
2-(3,4-Dihydroxyphenyl)acetic acid undergoes various chemical reactions, including:
Oxidation: This compound can be oxidized to form quinones.
Reduction: It can be reduced to form catechols.
Substitution: It can undergo electrophilic aromatic substitution reactions due to the presence of hydroxyl groups on the aromatic ring.
Common Reagents and Conditions
Common reagents used in these reactions include oxidizing agents like hydrogen peroxide and reducing agents like sodium borohydride. The reactions typically occur under mild conditions, such as room temperature and neutral pH .
Major Products Formed
The major products formed from these reactions include quinones, catechols, and various substituted phenylacetic acids .
Scientific Research Applications
2-(3,4-Dihydroxyphenyl)acetic acid has numerous applications in scientific research:
Chemistry: It is used as a precursor in the synthesis of various organic compounds.
Biology: It serves as a biomarker for studying the metabolism of dopamine and other neurotransmitters.
Medicine: It is used in the development of drugs targeting the dopaminergic system, particularly for treating neurological disorders.
Industry: It is utilized in the production of antioxidants and other bioactive compounds
Mechanism of Action
The mechanism of action of 2-(3,4-dihydroxyphenyl)acetic acid involves its role as a metabolite of dopamine. It is produced through the enzymatic degradation of dopamine by monoamine oxidase (MAO) and catechol-O-methyl transferase (COMT). This compound acts as a biomarker for dopamine metabolism and is involved in various biochemical pathways related to neurotransmitter regulation .
Comparison with Similar Compounds
Similar Compounds
Homovanillic acid: Another metabolite of dopamine, but with different enzymatic pathways.
3-Methoxytyramine: A metabolite of dopamine that is further degraded to homovanillic acid.
Phenylacetic acid: A structural derivative of 2-(3,4-dihydroxyphenyl)acetic acid.
Uniqueness
This compound is unique due to its specific role in the dopaminergic system and its involvement in the metabolism of dopamine. Its presence as a biomarker for dopamine metabolism makes it a valuable compound in neurological research .
Properties
IUPAC Name |
2-(3,4-dihydroxyphenyl)acetic acid | |
---|---|---|
Source | PubChem | |
URL | https://pubchem.ncbi.nlm.nih.gov | |
Description | Data deposited in or computed by PubChem | |
InChI |
InChI=1S/C8H8O4/c9-6-2-1-5(3-7(6)10)4-8(11)12/h1-3,9-10H,4H2,(H,11,12)/i8+1,11+2,12+2 | |
Source | PubChem | |
URL | https://pubchem.ncbi.nlm.nih.gov | |
Description | Data deposited in or computed by PubChem | |
InChI Key |
CFFZDZCDUFSOFZ-SWDPYBNISA-N | |
Source | PubChem | |
URL | https://pubchem.ncbi.nlm.nih.gov | |
Description | Data deposited in or computed by PubChem | |
Canonical SMILES |
C1=CC(=C(C=C1CC(=O)O)O)O | |
Source | PubChem | |
URL | https://pubchem.ncbi.nlm.nih.gov | |
Description | Data deposited in or computed by PubChem | |
Isomeric SMILES |
C1=CC(=C(C=C1C[13C](=[18O])[18OH])O)O | |
Source | PubChem | |
URL | https://pubchem.ncbi.nlm.nih.gov | |
Description | Data deposited in or computed by PubChem | |
Molecular Formula |
C8H8O4 | |
Source | PubChem | |
URL | https://pubchem.ncbi.nlm.nih.gov | |
Description | Data deposited in or computed by PubChem | |
Molecular Weight |
173.14 g/mol | |
Source | PubChem | |
URL | https://pubchem.ncbi.nlm.nih.gov | |
Description | Data deposited in or computed by PubChem | |
Synthesis routes and methods I
Procedure details
Synthesis routes and methods II
Procedure details
Disclaimer and Information on In-Vitro Research Products
Please be aware that all articles and product information presented on BenchChem are intended solely for informational purposes. The products available for purchase on BenchChem are specifically designed for in-vitro studies, which are conducted outside of living organisms. In-vitro studies, derived from the Latin term "in glass," involve experiments performed in controlled laboratory settings using cells or tissues. It is important to note that these products are not categorized as medicines or drugs, and they have not received approval from the FDA for the prevention, treatment, or cure of any medical condition, ailment, or disease. We must emphasize that any form of bodily introduction of these products into humans or animals is strictly prohibited by law. It is essential to adhere to these guidelines to ensure compliance with legal and ethical standards in research and experimentation.