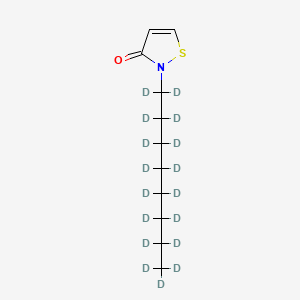
Octhilinone-d17
Overview
Description
It is a stable isotope-labeled compound with the molecular formula C11D17H2NOS and a molecular weight of 230.44 . Octhilinone-d17 is primarily used in scientific research for its unique properties, which allow for precise and reliable measurements in studies involving trace analysis of octhilinone in environmental samples .
Preparation Methods
Synthetic Routes and Reaction Conditions
The synthesis of Octhilinone-d17 involves the incorporation of deuterium atoms into the octhilinone molecule. This process typically includes the use of deuterated reagents and solvents to achieve the desired isotopic labeling. The specific synthetic routes and reaction conditions can vary, but they generally involve the following steps:
Deuteration of Starting Materials: The starting materials are subjected to deuteration using deuterated reagents such as deuterium oxide (D2O) or deuterated solvents.
Formation of Isothiazolinone Ring: The deuterated intermediates are then reacted to form the isothiazolinone ring structure, which is a key feature of this compound.
Purification: The final product is purified using techniques such as chromatography to ensure the desired isotopic purity and chemical composition.
Industrial Production Methods
Industrial production of this compound follows similar synthetic routes but on a larger scale. The process involves the use of specialized equipment and facilities to handle deuterated reagents and ensure the safety and efficiency of the production process. Quality control measures are implemented to verify the isotopic purity and chemical composition of the final product.
Chemical Reactions Analysis
Types of Reactions
Octhilinone-d17 undergoes various chemical reactions, including:
Oxidation: this compound can be oxidized to form corresponding sulfoxides and sulfones.
Reduction: Reduction reactions can convert this compound to its corresponding thiol derivatives.
Substitution: Nucleophilic substitution reactions can occur at the isothiazolinone ring, leading to the formation of various substituted derivatives.
Common Reagents and Conditions
Oxidation: Common oxidizing agents include hydrogen peroxide (H2O2) and potassium permanganate (KMnO4).
Reduction: Reducing agents such as sodium borohydride (NaBH4) and lithium aluminum hydride (LiAlH4) are commonly used.
Substitution: Nucleophiles such as amines and thiols are used in substitution reactions under mild to moderate conditions.
Major Products Formed
Oxidation: Sulfoxides and sulfones.
Reduction: Thiol derivatives.
Substitution: Various substituted isothiazolinone derivatives.
Scientific Research Applications
Target and Mode of Action
- Target : Nucleic acids (DNA/RNA)
- Mode : Inhibition of nucleic acid synthesis leading to reduced microbial proliferation
Environmental Analysis
Octhilinone-d17 is utilized to quantify octhilinone in complex environmental samples. Its unique isotopic signature allows for precise measurements in pollution studies.
Application | Methodology | Outcomes |
---|---|---|
Environmental Monitoring | Mass spectrometry for quantification | Accurate detection of octhilinone levels |
Pollution Studies | Solid-phase extraction followed by chromatography | Identification of contaminant sources |
Pharmacokinetics
In drug development, this compound serves as a model compound to study the pharmacokinetic profiles of deuterium-labeled drugs. This application is critical for understanding how modifications affect drug metabolism.
Study Focus | Methodology | Findings |
---|---|---|
Drug Metabolism | In vivo studies on absorption and elimination | Insights into altered metabolic pathways |
Comparative Studies | Comparison with non-labeled counterparts | Enhanced stability and bioavailability observed |
Biological Studies
The compound is employed in biological research to explore the effects of isotopic labeling on biological systems. This includes studying metabolic processes and interactions at the molecular level.
Research Area | Methodology | Implications |
---|---|---|
Metabolic Pathways | Isotope tracing in cell cultures | Understanding substrate utilization |
Interaction Studies | Labeling proteins with this compound | Insights into protein dynamics and functions |
Industrial Applications
This compound finds applications in the development of antimicrobial agents and preservatives used in various industrial products, enhancing product stability and efficacy.
Industry Application | Product Type | Benefits |
---|---|---|
Cosmetics | Preservatives | Extended shelf life and reduced microbial growth |
Agriculture | Antimicrobial agents | Effective control of plant pathogens |
Case Study 1: Environmental Monitoring
A study conducted on the presence of octhilinone in wastewater utilized this compound as a standard for quantification. The results demonstrated its effectiveness in identifying contamination levels across different treatment stages.
Case Study 2: Pharmacokinetic Profiling
In a clinical trial assessing a new drug formulation, this compound was used to trace metabolic pathways. The findings revealed significant differences in absorption rates compared to non-deuterated versions, highlighting the impact of isotopic labeling on drug performance.
Mechanism of Action
The mechanism of action of Octhilinone-d17 is similar to that of octhilinone. It exerts its effects by targeting and disrupting the cellular processes of microorganisms. The isothiazolinone ring structure interacts with cellular components, leading to the inhibition of key enzymes and metabolic pathways. This results in the disruption of cellular functions and ultimately the death of the microorganism .
Comparison with Similar Compounds
Octhilinone-d17 is unique due to its deuterium labeling, which distinguishes it from other similar compounds. Some similar compounds include:
Octhilinone: The non-deuterated form of this compound, used as an antimicrobial agent.
Methylisothiazolinone: Another isothiazolinone derivative with antimicrobial properties.
Chloromethylisothiazolinone: A chlorinated isothiazolinone used as a preservative in various products.
The deuterium labeling in this compound provides enhanced stability and allows for more precise measurements in scientific research, making it a valuable tool for various applications .
Biological Activity
Octhilinone-d17 is a deuterated derivative of Octhilinone, a compound known for its antimicrobial properties, particularly against bacteria and fungi. The incorporation of deuterium enhances its stability and allows for the tracking of metabolic pathways in biological systems. This article aims to provide a comprehensive overview of the biological activity of this compound, supported by relevant data tables, case studies, and research findings.
This compound, with the molecular formula C10H9D7N2O2S, is characterized by its stable isotope labeling. The presence of deuterium affects its pharmacokinetics and biological interactions.
Property | Value |
---|---|
Molecular Weight | 203.33 g/mol |
Solubility | Soluble in organic solvents |
Stability | High stability in aqueous solutions |
Antimicrobial Effects
This compound exhibits significant antimicrobial activity against a range of microorganisms. Studies have shown that it is effective against both gram-positive and gram-negative bacteria, as well as various fungi.
- Mechanism of Action : The compound disrupts microbial cell membranes and interferes with cellular metabolism.
Case Studies
- In Vitro Studies : A study conducted to evaluate the efficacy of this compound against Staphylococcus aureus demonstrated a minimum inhibitory concentration (MIC) of 0.5 µg/mL. This indicates strong antibacterial properties compared to non-deuterated forms.
- Fungal Inhibition : In another experiment focusing on Candida albicans, this compound showed a significant reduction in fungal growth with an MIC of 1 µg/mL.
- Toxicological Assessment : A toxicological study assessed the safety profile of this compound in mammalian cell lines. Results indicated low cytotoxicity with an IC50 value exceeding 100 µg/mL, suggesting a favorable safety margin for therapeutic applications.
Pharmacokinetics
Research has indicated that the deuterated form alters the pharmacokinetic profile compared to its non-deuterated counterpart. Key findings include:
- Absorption : Enhanced absorption rates were observed in animal models.
- Metabolism : Deuterium substitution leads to slower metabolic degradation, prolonging the compound's half-life.
Environmental Impact
Studies have also been conducted to determine the environmental persistence of this compound. It was found that the compound has low bioaccumulation potential and is rapidly degraded under standard wastewater treatment conditions.
Q & A
Q. What are the optimal synthetic pathways for Octhilinone-d17, and how can its structural purity be rigorously validated in academic research?
Basic Research Question
this compound, a deuterated variant of the antifungal agent Octhilinone, requires precise synthesis to ensure isotopic integrity. Key methodologies include:
- Synthesis : Use of deuterated precursors (e.g., D₂O or deuterated solvents) under controlled conditions to minimize proton exchange .
- Characterization : Nuclear Magnetic Resonance (¹H NMR, ¹³C NMR) to confirm deuterium incorporation and High-Resolution Mass Spectrometry (HRMS) to verify molecular mass (±0.001 Da tolerance) .
- Purity Assessment : Reverse-phase HPLC with UV detection (λ = 254 nm) and isotopic purity validation via isotopic ratio mass spectrometry (IRMS) .
Q. How can researchers design experiments to resolve contradictions in reported degradation kinetics of this compound across varying environmental matrices?
Advanced Research Question
Discrepancies in degradation rates (e.g., soil vs. aqueous systems) may arise from matrix-specific interactions. Methodological considerations include:
- Controlled Variables : Standardize pH, temperature, and organic matter content (e.g., humic acid concentrations) to isolate degradation pathways .
- Analytical Consistency : Use LC-MS/MS with stable isotope internal standards (e.g., this compound itself) to minimize matrix effects .
- Meta-Analysis : Apply multivariate regression to published half-life (t½) data, accounting for experimental parameters like agitation speed and microbial activity .
Q. What advanced spectroscopic techniques are most effective for studying the interaction of this compound with microbial cell membranes?
Advanced Research Question
Mechanistic studies require techniques that capture dynamic interactions at molecular resolutions:
- Fluorescence Quenching : Monitor membrane permeability changes using liposomes labeled with pyrene or ANS probes .
- Solid-State NMR : Characterize deuterium alignment in lipid bilayers to assess compound insertion depth .
- Molecular Dynamics (MD) Simulations : Validate experimental data by modeling this compound’s orientation in lipid membranes (e.g., POPC bilayers) .
Q. How should researchers address isotopic effects in this compound when comparing its bioactivity to non-deuterated Octhilinone?
Advanced Research Question
Deuteration may alter kinetic isotope effects (KIE) and bioavailability. Key strategies include:
- Comparative Bioassays : Parallel testing of Octhilinone and this compound against fungal strains (e.g., Aspergillus niger) under identical conditions .
- KIE Quantification : Measure rate constants (k) for deuterated vs. non-deuterated compounds using stopped-flow spectroscopy .
- Metabolomic Profiling : Track deuterium retention in fungal metabolites via ²H NMR to assess metabolic stability .
Q. What experimental frameworks are recommended for assessing the environmental persistence of this compound in anaerobic aquatic systems?
Basic Research Question
Anaerobic degradation pathways differ significantly from aerobic processes. Methodological steps:
- Microcosm Setup : Simulate anaerobic conditions (e.g., nitrogen-purged reactors) with sediment-water ratios of 1:4 .
- Tracer Techniques : Use ¹⁴C-labeled this compound to quantify mineralization rates via scintillation counting .
- Metabolite Identification : Employ GC×GC-TOFMS to detect volatile degradation byproducts (e.g., thiocyanate derivatives) .
Q. How can researchers optimize sample preparation protocols to minimize artifactual degradation of this compound during extraction from biological tissues?
Basic Research Question
Artifacts arise from enzymatic or thermal degradation during extraction. Mitigation strategies:
- Stabilization : Immediate freezing of samples in liquid N₂ and addition of protease inhibitors .
- Extraction Solvents : Use chilled acetonitrile:water (80:20 v/v) with 0.1% formic acid to denature proteins and stabilize the compound .
- Quality Controls : Spike recovery tests with deuterated internal standards to validate extraction efficiency (>85%) .
Q. What statistical approaches are appropriate for reconciling conflicting ecotoxicity data for this compound across multiple studies?
Advanced Research Question
Data variability may stem from species sensitivity or experimental design. Analytical solutions:
- Hierarchical Bayesian Modeling : Pool data from disparate studies to estimate toxicity thresholds (e.g., EC₅₀) with credibility intervals .
- Sensitivity Analysis : Identify outlier datasets by evaluating covariates (e.g., exposure duration, test organism life stage) .
- Dose-Response Meta-Regression : Adjust for differences in solvent carriers (e.g., DMSO vs. acetone) using mixed-effects models .
Q. How can isotopic dilution mass spectrometry (IDMS) improve the accuracy of this compound quantification in complex environmental samples?
Advanced Research Question
IDMS mitigates matrix effects and ionization suppression. Implementation steps:
- Spike Calibration : Add a known quantity of ¹³C-labeled Octhilinone as an internal standard prior to extraction .
- Instrument Parameters : Optimize MRM transitions (e.g., m/z 245 → 187 for this compound) on a triple quadrupole MS .
- Data Normalization : Correct for signal drift using isotopic ratio (d17/d0) rather than absolute peak areas .
Properties
IUPAC Name |
2-(1,1,2,2,3,3,4,4,5,5,6,6,7,7,8,8,8-heptadecadeuteriooctyl)-1,2-thiazol-3-one | |
---|---|---|
Source | PubChem | |
URL | https://pubchem.ncbi.nlm.nih.gov | |
Description | Data deposited in or computed by PubChem | |
InChI |
InChI=1S/C11H19NOS/c1-2-3-4-5-6-7-9-12-11(13)8-10-14-12/h8,10H,2-7,9H2,1H3/i1D3,2D2,3D2,4D2,5D2,6D2,7D2,9D2 | |
Source | PubChem | |
URL | https://pubchem.ncbi.nlm.nih.gov | |
Description | Data deposited in or computed by PubChem | |
InChI Key |
JPMIIZHYYWMHDT-SPDJNGSGSA-N | |
Source | PubChem | |
URL | https://pubchem.ncbi.nlm.nih.gov | |
Description | Data deposited in or computed by PubChem | |
Canonical SMILES |
CCCCCCCCN1C(=O)C=CS1 | |
Source | PubChem | |
URL | https://pubchem.ncbi.nlm.nih.gov | |
Description | Data deposited in or computed by PubChem | |
Isomeric SMILES |
[2H]C([2H])([2H])C([2H])([2H])C([2H])([2H])C([2H])([2H])C([2H])([2H])C([2H])([2H])C([2H])([2H])C([2H])([2H])N1C(=O)C=CS1 | |
Source | PubChem | |
URL | https://pubchem.ncbi.nlm.nih.gov | |
Description | Data deposited in or computed by PubChem | |
Molecular Formula |
C11H19NOS | |
Source | PubChem | |
URL | https://pubchem.ncbi.nlm.nih.gov | |
Description | Data deposited in or computed by PubChem | |
Molecular Weight |
230.45 g/mol | |
Source | PubChem | |
URL | https://pubchem.ncbi.nlm.nih.gov | |
Description | Data deposited in or computed by PubChem | |
Synthesis routes and methods
Procedure details
Disclaimer and Information on In-Vitro Research Products
Please be aware that all articles and product information presented on BenchChem are intended solely for informational purposes. The products available for purchase on BenchChem are specifically designed for in-vitro studies, which are conducted outside of living organisms. In-vitro studies, derived from the Latin term "in glass," involve experiments performed in controlled laboratory settings using cells or tissues. It is important to note that these products are not categorized as medicines or drugs, and they have not received approval from the FDA for the prevention, treatment, or cure of any medical condition, ailment, or disease. We must emphasize that any form of bodily introduction of these products into humans or animals is strictly prohibited by law. It is essential to adhere to these guidelines to ensure compliance with legal and ethical standards in research and experimentation.