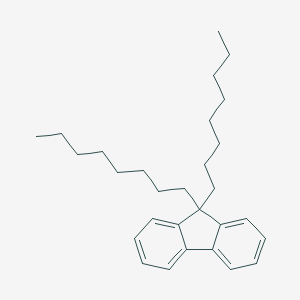
9,9-Di-n-octylfluorene
Overview
Description
9,9-Di-n-octylfluorene is a derivative of fluorene, characterized by the substitution of two octyl groups at the 9-position of the fluorene core. This compound is known for its high photoluminescence efficiency and chemical stability, making it a valuable material in the field of optoelectronics, particularly in polymer light-emitting diodes (PLEDs) and organic light-emitting diodes (OLEDs) .
Scientific Research Applications
9,9-Di-n-octylfluorene has a wide range of applications in scientific research:
Optoelectronics: Used in the fabrication of PLEDs and OLEDs due to its high photoluminescence efficiency and stability.
Material Science: Employed in the synthesis of diblock copolymers for enhanced electron transport properties.
Nanotechnology: Utilized to disperse single-walled carbon nanotubes (SWCNTs) for the fabrication of field-effect transistors (FETs).
Biomedical Research: Investigated for potential use in biosensors and imaging applications due to its fluorescent properties.
Mechanism of Action
Target of Action
9,9-Di-n-octylfluorene, also known as Poly (9,9-di-n-octylfluorenyl-2,7-diyl) (PFO), is a blue light-emitting fluorescent polymer . Its primary target is the optoelectronic devices where it forms an emissive layer .
Mode of Action
The mode of action of this compound involves the formation of an ordered β conformation both in solution and film . This ordered structure is crucial for preparing high-efficiency optoelectronic devices . The β-phase can be generated by introducing an ambipolar unit on a polyfluorene backbone .
Biochemical Pathways
The biochemical pathways of this compound involve the transformation of chain structure and β conformation from the solution to the film . This transformation process is influenced by the molecular weight and involves three stages . The formation of the β-phase can be prompted after spin-coating without gelling in a poor solvent, exposure to tetrahydrofuran vapor, cooling to liquid-N2 temperature, and reheating .
Result of Action
The result of the action of this compound is the emission of blue light with high electroluminescence quantum yield . The photoluminescence spectrum of PFO in a dilute solid-state solution in polystyrene redshifts by 320 meV over 7.4 GPa, while that of a PFO thin film redshifts 460 meV over a comparable pressure range .
Action Environment
The action environment of this compound includes the conditions under which the β-phase is formed. This includes the solvent environment, temperature changes, and the presence of ambipolar moieties . The formation of the β-phase can be influenced by environmental factors such as solvent type and temperature .
Safety and Hazards
Future Directions
Preparation Methods
Synthetic Routes and Reaction Conditions: The synthesis of 9,9-Di-n-octylfluorene typically involves the alkylation of fluorene. One common method is the Friedel-Crafts alkylation, where fluorene reacts with octyl chloride in the presence of a Lewis acid catalyst such as aluminum chloride. The reaction proceeds under anhydrous conditions to yield this compound .
Industrial Production Methods: In industrial settings, the production of this compound may involve continuous flow processes to enhance efficiency and scalability. The use of advanced catalytic systems and optimized reaction conditions ensures high yield and purity of the final product .
Chemical Reactions Analysis
Types of Reactions: 9,9-Di-n-octylfluorene undergoes various chemical reactions, including:
Oxidation: The compound can be oxidized to form fluorenone derivatives.
Substitution: Electrophilic substitution reactions can introduce different functional groups onto the fluorene core.
Polymerization: It can be polymerized to form polyfluorene derivatives, which are used in optoelectronic applications.
Common Reagents and Conditions:
Oxidation: Common oxidizing agents include potassium permanganate and chromium trioxide.
Substitution: Reagents such as halogens and nitrating agents are used under controlled conditions to achieve selective substitution.
Major Products:
Oxidation: Fluorenone derivatives.
Substitution: Halogenated or nitrated fluorenes.
Polymerization: Poly(this compound) (PFO), a blue light-emitting polymer.
Comparison with Similar Compounds
Poly(9,9-di-n-octylfluorenyl-2,7-diyl) (PFO): A polymer derivative with similar optoelectronic properties.
Poly(9,9-di-n-octylfluorene-alt-benzothiadiazole) (F8BT): A green-emitting polyfluorene derivative with good electron transport properties.
Poly(9,9-bis(2-ethylhexyl)-9H-fluorene-2,7-diyl): Another polyfluorene derivative used in optoelectronics.
Uniqueness: this compound stands out due to its high chemical stability and efficient photoluminescence, making it a preferred choice for blue light-emitting applications. Its ability to form stable polymers and copolymers further enhances its versatility in various scientific and industrial applications .
Properties
IUPAC Name |
9,9-dioctylfluorene | |
---|---|---|
Source | PubChem | |
URL | https://pubchem.ncbi.nlm.nih.gov | |
Description | Data deposited in or computed by PubChem | |
InChI |
InChI=1S/C29H42/c1-3-5-7-9-11-17-23-29(24-18-12-10-8-6-4-2)27-21-15-13-19-25(27)26-20-14-16-22-28(26)29/h13-16,19-22H,3-12,17-18,23-24H2,1-2H3 | |
Source | PubChem | |
URL | https://pubchem.ncbi.nlm.nih.gov | |
Description | Data deposited in or computed by PubChem | |
InChI Key |
RXACYPFGPNTUNV-UHFFFAOYSA-N | |
Source | PubChem | |
URL | https://pubchem.ncbi.nlm.nih.gov | |
Description | Data deposited in or computed by PubChem | |
Canonical SMILES |
CCCCCCCCC1(C2=CC=CC=C2C3=CC=CC=C31)CCCCCCCC | |
Source | PubChem | |
URL | https://pubchem.ncbi.nlm.nih.gov | |
Description | Data deposited in or computed by PubChem | |
Molecular Formula |
C29H42 | |
Source | PubChem | |
URL | https://pubchem.ncbi.nlm.nih.gov | |
Description | Data deposited in or computed by PubChem | |
Related CAS |
123864-00-6 | |
Record name | Poly(9,9-dioctylfluorene) | |
Source | CAS Common Chemistry | |
URL | https://commonchemistry.cas.org/detail?cas_rn=123864-00-6 | |
Description | CAS Common Chemistry is an open community resource for accessing chemical information. Nearly 500,000 chemical substances from CAS REGISTRY cover areas of community interest, including common and frequently regulated chemicals, and those relevant to high school and undergraduate chemistry classes. This chemical information, curated by our expert scientists, is provided in alignment with our mission as a division of the American Chemical Society. | |
Explanation | The data from CAS Common Chemistry is provided under a CC-BY-NC 4.0 license, unless otherwise stated. | |
DSSTOX Substance ID |
DTXSID70584145 | |
Record name | 9,9-Dioctyl-9H-fluorene | |
Source | EPA DSSTox | |
URL | https://comptox.epa.gov/dashboard/DTXSID70584145 | |
Description | DSSTox provides a high quality public chemistry resource for supporting improved predictive toxicology. | |
Molecular Weight |
390.6 g/mol | |
Source | PubChem | |
URL | https://pubchem.ncbi.nlm.nih.gov | |
Description | Data deposited in or computed by PubChem | |
CAS No. |
123863-99-0 | |
Record name | 9,9-Dioctyl-9H-fluorene | |
Source | CAS Common Chemistry | |
URL | https://commonchemistry.cas.org/detail?cas_rn=123863-99-0 | |
Description | CAS Common Chemistry is an open community resource for accessing chemical information. Nearly 500,000 chemical substances from CAS REGISTRY cover areas of community interest, including common and frequently regulated chemicals, and those relevant to high school and undergraduate chemistry classes. This chemical information, curated by our expert scientists, is provided in alignment with our mission as a division of the American Chemical Society. | |
Explanation | The data from CAS Common Chemistry is provided under a CC-BY-NC 4.0 license, unless otherwise stated. | |
Record name | 9,9-Dioctyl-9H-fluorene | |
Source | EPA DSSTox | |
URL | https://comptox.epa.gov/dashboard/DTXSID70584145 | |
Description | DSSTox provides a high quality public chemistry resource for supporting improved predictive toxicology. | |
Synthesis routes and methods
Procedure details
Retrosynthesis Analysis
AI-Powered Synthesis Planning: Our tool employs the Template_relevance Pistachio, Template_relevance Bkms_metabolic, Template_relevance Pistachio_ringbreaker, Template_relevance Reaxys, Template_relevance Reaxys_biocatalysis model, leveraging a vast database of chemical reactions to predict feasible synthetic routes.
One-Step Synthesis Focus: Specifically designed for one-step synthesis, it provides concise and direct routes for your target compounds, streamlining the synthesis process.
Accurate Predictions: Utilizing the extensive PISTACHIO, BKMS_METABOLIC, PISTACHIO_RINGBREAKER, REAXYS, REAXYS_BIOCATALYSIS database, our tool offers high-accuracy predictions, reflecting the latest in chemical research and data.
Strategy Settings
Precursor scoring | Relevance Heuristic |
---|---|
Min. plausibility | 0.01 |
Model | Template_relevance |
Template Set | Pistachio/Bkms_metabolic/Pistachio_ringbreaker/Reaxys/Reaxys_biocatalysis |
Top-N result to add to graph | 6 |
Feasible Synthetic Routes
Q1: What is the molecular formula and weight of 9,9-Di-n-octylfluorene?
A1: this compound has the molecular formula C29H42 and a molecular weight of 390.66 g/mol.
Q2: What spectroscopic techniques are commonly used to characterize this compound and its polymers?
A2: Researchers frequently utilize a range of spectroscopic methods including:
- NMR Spectroscopy: This technique is crucial for determining the structure and purity of this compound monomers and polymers. [, , , , , ]
- UV-Vis Spectroscopy: This method is employed to analyze the absorption and transmission properties of this compound-based materials, particularly their conjugated systems. [, , , , , , , , ]
- Photoluminescence Spectroscopy: This technique helps researchers understand the light emission characteristics of this compound-based materials, essential for applications like LEDs and sensors. [, , , , , , , , , , , , , ]
- Raman Spectroscopy: This tool is particularly useful in investigating the morphology and chain conformation of this compound-based polymers in thin films. [, , , ]
Q3: How does the molecular weight of poly(this compound-alt-benzothiadiazole) (F8BT) affect its thin-film morphology?
A3: Studies reveal that higher molecular weight F8BT tends to form films with polymer chains lying preferentially in the plane of the substrate, leading to measurable optical anisotropy. [, ]
Q4: Does annealing affect the properties of F8BT thin films?
A4: Yes, annealing significantly influences the properties of F8BT films. It leads to a more planar conformation of polymer chains, increased optical anisotropy, and changes in absorption and emission characteristics. [, , , ] Annealing also affects electron mobility and triplet generation dynamics within the films. [, ]
Q5: How does the choice of substrate affect the conformation of F8BT chains at the interface?
A5: Research using Raman spectroscopy shows that F8BT chains adopt a more planar conformation near the interface with various substrates (quartz, crosslinked benzocyclobutene, polyvinylphenol, PEDOT:PSS) compared to the bulk of the film. []
Q6: Does this compound itself exhibit catalytic properties?
A6: The provided research focuses on the use of this compound as a building block for polymeric materials used in organic electronics. Its direct catalytic properties are not discussed.
Q7: Have computational methods been used to study this compound-based materials?
A7: Yes, computational chemistry plays a role in understanding these materials. For instance, quantum-chemical calculations have been employed to:
- Investigate the electronic properties of F8BT copolymers, revealing the localization of molecular orbitals and charge-transfer characteristics of excitons. []
- Analyze the electron transport mechanisms in F8BT, comparing intrachain and interchain transport efficiency. []
- Study the impact of polymer packing structure on photoinduced triplet generation and dynamics in F8BT. []
- Molecular mechanics simulations have been used to rationalize the propensity of spiro-oligo(fluorene)s to thermally activated crystallization. []
Q8: How does the structure of polyfluorene copolymers influence their optical properties, as revealed by computational studies?
A8: Quantum-chemical calculations on polyfluorene copolymers with varying benzothiadiazole content show that a lower benzothiadiazole ratio leads to a reduced oscillator strength of the lowest optical transition and an increased charge-transfer character of the exciton. []
Q9: What is the role of the bridging unit in benzimidazole-fluorene copolymers?
A10: Research on benzimidazole-fluorene copolymers demonstrates that incorporating vinyl and ethynyl bridges helps separate benzimidazole and fluorene units while retaining conjugation. [] This separation allows researchers to differentiate between steric and electronic contributions to band gap changes upon doping. []
Q10: How can the stability of this compound-based polymers be enhanced?
A10: While the provided research doesn't explicitly focus on formulation strategies, it highlights that:
- Spiro-linked oligofluorenes exhibit enhanced thermal stability against crystallization compared to poly(2,7-(this compound)). []
- The presence of a poly(this compound-alt-(1,4-phenylene-(4-sec-butylphenyl)imino)-1,4-phenylene) (TFB) layer between graphene and PMMA can act as a protector during graphene transfer, improving device stability and performance in OLEDs. []
Q11: Are there methods to improve the thickness uniformity of inkjet-printed poly(this compound) films?
A12: Researchers have successfully improved thickness uniformity by using solvent mixtures during inkjet printing. Employing a solvent with high volatility and low viscosity alongside a solvent with low volatility and high viscosity can lead to more uniform films by influencing Marangoni flow during the drying process. []
Q12: What SHE considerations are relevant to this compound research?
A12: The provided research primarily focuses on the material science and optoelectronic applications of this compound-based materials. SHE regulations, while crucial for any chemical research, are not explicitly discussed in these papers.
Q13: Can you provide details on the PK/PD, in vitro and in vivo efficacy, resistance, toxicology, drug delivery, biomarkers, or environmental impact of this compound?
A13: The provided research focuses on the material science and optoelectronic applications of this compound. Questions related to pharmacology, toxicology, or environmental impact are outside the scope of these studies.
Q14: How is the β phase content in polyfluorenes typically determined?
A15: The β phase content in polyfluorenes is often determined through analysis of their absorption and emission spectra. The presence of characteristic peaks associated with the β phase allows for qualitative and quantitative assessment. [, ]
Q15: What solvents are commonly used for processing this compound and its polymers?
A15: The research highlights the use of various solvents, including:
- Chlorobenzene: Often used for solution processing of polyfluorene-based materials. [, ]
- Toluene: Employed in the synthesis and processing of polyfluorenes, as well as in studies exploring β phase formation. [, , , ]
- MCH (methylcyclohexane): Utilized in studies investigating the aggregation behavior of polyfluorenes in solution. []
- Mixtures of solvents with differing properties (volatility, viscosity) are also used to control film morphology during inkjet printing. [, ]
Disclaimer and Information on In-Vitro Research Products
Please be aware that all articles and product information presented on BenchChem are intended solely for informational purposes. The products available for purchase on BenchChem are specifically designed for in-vitro studies, which are conducted outside of living organisms. In-vitro studies, derived from the Latin term "in glass," involve experiments performed in controlled laboratory settings using cells or tissues. It is important to note that these products are not categorized as medicines or drugs, and they have not received approval from the FDA for the prevention, treatment, or cure of any medical condition, ailment, or disease. We must emphasize that any form of bodily introduction of these products into humans or animals is strictly prohibited by law. It is essential to adhere to these guidelines to ensure compliance with legal and ethical standards in research and experimentation.