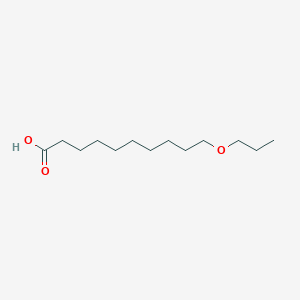
10-Propoxydecanoic acid
Overview
Description
O-11 is an analog of the fully saturated, 14-carbon fatty acid myristic acid, in which the methylene group at position 11 is replaced with oxygen. This compound is highly effective and selective at killing Trypanosoma brucei, the protozoan parasite responsible for African sleeping sickness . The toxic effects of O-11 appear to be caused by its ability to inhibit the incorporation of a single myristate into the glycosylphosphatidylinositol (GPI) anchor of the variant surface glycoprotein (VSG), a protein critical for evading the host immune response .
Mechanism of Action
Target of Action
10-Propoxydecanoic acid, also known as compound 011, is a toxic myristate analog . The primary target of this compound is the Trypanosoma brucei , the protozoan parasite responsible for African sleeping sickness .
Mode of Action
The compound interacts with its target by inhibiting the incorporation of a single myristate into the GPI anchor of the variant surface glycoprotein (VSG), a protein critical for evading the host immune response .
Biochemical Pathways
It is known that the compound disrupts the normal functioning of the trypanosoma brucei parasite by interfering with the myristate incorporation process .
Pharmacokinetics
It is known that the compound exhibits an ic50 value of 14μm on ketri 243 , indicating its potency.
Result of Action
The result of the action of this compound is the effective killing of the Trypanosoma brucei parasite. It exhibits a lethal dose 50 (LD50) of less than 1 μM in a cell culture assay .
Action Environment
It is known that the compound is stored at room temperature in continental us , which may suggest that it is stable under normal environmental conditions.
Biochemical Analysis
Biochemical Properties
10-Propoxydecanoic acid has been found to be highly effective and selective at killing Trypanosoma brucei, the protozoan parasite responsible for African sleeping sickness . The toxic effects of this compound appear to be caused by its ability to inhibit the incorporation of a single myristate into the GPI anchor of the variant surface glycoprotein (VSG), a protein critical for evading the host immune response .
Cellular Effects
In cellular assays, this compound has shown an LD50 of less than 1 μM, indicating its potent cytotoxicity . It exhibits essentially no anti-fungal activity when assayed using C. neoformans, but does have a minor inhibitory effect on HIV-1 replication in T-lymphocytes .
Molecular Mechanism
The molecular mechanism of action of this compound involves the inhibition of the incorporation of a single myristate into the GPI anchor of the variant surface glycoprotein (VSG) . This inhibition disrupts the function of VSG, a protein critical for evading the host immune response, leading to the death of Trypanosoma brucei .
Preparation Methods
The synthesis of O-11 involves the modification of myristic acid. The methylene group at position 11 is replaced with an oxygen atom. This can be achieved through various synthetic routes, including:
Oxidation of Myristic Acid: Myristic acid can be oxidized to introduce an oxygen atom at the desired position.
Transmetallation: This involves the reaction of an electropositive metal with a halogen-substituted hydrocarbon to form the desired compound.
Metathesis: This synthetic route involves the reaction of an organometallic compound with a binary halide.
Chemical Reactions Analysis
O-11 undergoes various chemical reactions, including:
Oxidation: O-11 can be further oxidized to form different products depending on the reaction conditions.
Reduction: Reduction reactions can convert O-11 back to its precursor forms.
Substitution: The oxygen atom in O-11 can be substituted with other functional groups under specific conditions.
Common reagents used in these reactions include oxidizing agents like potassium permanganate and reducing agents like lithium aluminum hydride. The major products formed from these reactions depend on the specific reagents and conditions used.
Scientific Research Applications
O-11 has several scientific research applications, including:
Chemistry: O-11 is used as a model compound to study the effects of oxygen substitution in fatty acids.
Industry: O-11 can be used in the development of new drugs and treatments for parasitic infections.
Comparison with Similar Compounds
O-11 is unique due to the oxygen substitution at position 11 of the myristic acid molecule. Similar compounds include:
Myristic Acid: The parent compound of O-11, which lacks the oxygen substitution.
Lauric Acid: A 12-carbon fatty acid with similar properties but without the oxygen substitution.
Palmitic Acid: A 16-carbon fatty acid that shares some structural similarities with O-11.
The uniqueness of O-11 lies in its selective toxicity towards Trypanosoma brucei and its ability to inhibit the GPI anchor synthesis, which is not observed in the similar compounds listed above.
Properties
IUPAC Name |
10-propoxydecanoic acid | |
---|---|---|
Source | PubChem | |
URL | https://pubchem.ncbi.nlm.nih.gov | |
Description | Data deposited in or computed by PubChem | |
InChI |
InChI=1S/C13H26O3/c1-2-11-16-12-9-7-5-3-4-6-8-10-13(14)15/h2-12H2,1H3,(H,14,15) | |
Source | PubChem | |
URL | https://pubchem.ncbi.nlm.nih.gov | |
Description | Data deposited in or computed by PubChem | |
InChI Key |
HSRAJJQTYQHRSA-UHFFFAOYSA-N | |
Source | PubChem | |
URL | https://pubchem.ncbi.nlm.nih.gov | |
Description | Data deposited in or computed by PubChem | |
Canonical SMILES |
CCCOCCCCCCCCCC(=O)O | |
Source | PubChem | |
URL | https://pubchem.ncbi.nlm.nih.gov | |
Description | Data deposited in or computed by PubChem | |
Molecular Formula |
C13H26O3 | |
Source | PubChem | |
URL | https://pubchem.ncbi.nlm.nih.gov | |
Description | Data deposited in or computed by PubChem | |
DSSTOX Substance ID |
DTXSID80922918 | |
Record name | 10-Propoxydecanoic acid | |
Source | EPA DSSTox | |
URL | https://comptox.epa.gov/dashboard/DTXSID80922918 | |
Description | DSSTox provides a high quality public chemistry resource for supporting improved predictive toxicology. | |
Molecular Weight |
230.34 g/mol | |
Source | PubChem | |
URL | https://pubchem.ncbi.nlm.nih.gov | |
Description | Data deposited in or computed by PubChem | |
CAS No. |
119290-00-5, 119290-12-9 | |
Record name | 10-Propoxydecanoic acid | |
Source | ChemIDplus | |
URL | https://pubchem.ncbi.nlm.nih.gov/substance/?source=chemidplus&sourceid=0119290005 | |
Description | ChemIDplus is a free, web search system that provides access to the structure and nomenclature authority files used for the identification of chemical substances cited in National Library of Medicine (NLM) databases, including the TOXNET system. | |
Record name | 10-(Propoxy)decanoyl-coenzyme A | |
Source | ChemIDplus | |
URL | https://pubchem.ncbi.nlm.nih.gov/substance/?source=chemidplus&sourceid=0119290129 | |
Description | ChemIDplus is a free, web search system that provides access to the structure and nomenclature authority files used for the identification of chemical substances cited in National Library of Medicine (NLM) databases, including the TOXNET system. | |
Record name | 10-Propoxydecanoic acid | |
Source | EPA DSSTox | |
URL | https://comptox.epa.gov/dashboard/DTXSID80922918 | |
Description | DSSTox provides a high quality public chemistry resource for supporting improved predictive toxicology. | |
Synthesis routes and methods
Procedure details
Retrosynthesis Analysis
AI-Powered Synthesis Planning: Our tool employs the Template_relevance Pistachio, Template_relevance Bkms_metabolic, Template_relevance Pistachio_ringbreaker, Template_relevance Reaxys, Template_relevance Reaxys_biocatalysis model, leveraging a vast database of chemical reactions to predict feasible synthetic routes.
One-Step Synthesis Focus: Specifically designed for one-step synthesis, it provides concise and direct routes for your target compounds, streamlining the synthesis process.
Accurate Predictions: Utilizing the extensive PISTACHIO, BKMS_METABOLIC, PISTACHIO_RINGBREAKER, REAXYS, REAXYS_BIOCATALYSIS database, our tool offers high-accuracy predictions, reflecting the latest in chemical research and data.
Strategy Settings
Precursor scoring | Relevance Heuristic |
---|---|
Min. plausibility | 0.01 |
Model | Template_relevance |
Template Set | Pistachio/Bkms_metabolic/Pistachio_ringbreaker/Reaxys/Reaxys_biocatalysis |
Top-N result to add to graph | 6 |
Feasible Synthetic Routes
Q1: What makes 10-propoxydecanoic acid a potential anti-parasitic agent?
A: this compound is a heteroatom-containing analog of myristic acid, a saturated fatty acid crucial for the biosynthesis of glycosylphosphatidylinositol (GPI) anchors. In the parasite Trypanosoma brucei, the causative agent of African sleeping sickness, the variant surface glycoprotein (VSG) relies on a GPI anchor containing exclusively myristate. [] this compound disrupts this process by acting as a myristate analog, interfering with GPI biosynthesis and ultimately impacting parasite viability. [, ]
Q2: How does the structure of this compound contribute to its activity against Trypanosoma brucei?
A: While similar in chain length to myristate, this compound exhibits a lower hydrophobicity due to the presence of an oxygen atom replacing a methylene group. [] Research suggests that the selectivity of fatty acid incorporation into the GPI anchor depends on chain length rather than hydrophobicity. [] Therefore, this compound can compete with myristate during GPI biosynthesis, leading to the formation of dysfunctional anchors and disrupting VSG expression in Trypanosoma brucei. [, ]
Q3: Is this compound selectively toxic towards parasites?
A: Studies have shown that this compound exhibits selective toxicity towards Trypanosoma brucei while demonstrating low toxicity in mammalian cells. [] This selectivity makes it a promising candidate for the development of novel anti-parasitic therapies. Further research exploring the detailed mechanisms of uptake, metabolism, and incorporation of this compound in both parasite and mammalian cells is crucial to understand and exploit this selective toxicity. []
Q4: Beyond Trypanosoma brucei, what other applications are being explored for this compound and similar myristate analogs?
A: Research indicates that myristate analogs, including this compound, can inhibit the replication of certain retroviruses like HIV-1 and Moloney murine leukemia virus (MoMLV). [, ] These viruses rely on the myristoylation of their gag polyprotein precursors for assembly, a process that can be disrupted by the incorporation of myristate analogs. [, ] This highlights the potential of myristate analogs as broad-spectrum antiviral agents, although further research is needed to optimize their efficacy and safety profiles.
Disclaimer and Information on In-Vitro Research Products
Please be aware that all articles and product information presented on BenchChem are intended solely for informational purposes. The products available for purchase on BenchChem are specifically designed for in-vitro studies, which are conducted outside of living organisms. In-vitro studies, derived from the Latin term "in glass," involve experiments performed in controlled laboratory settings using cells or tissues. It is important to note that these products are not categorized as medicines or drugs, and they have not received approval from the FDA for the prevention, treatment, or cure of any medical condition, ailment, or disease. We must emphasize that any form of bodily introduction of these products into humans or animals is strictly prohibited by law. It is essential to adhere to these guidelines to ensure compliance with legal and ethical standards in research and experimentation.